Evolutionarily conserved sperm factors, DCST1 and DCST2, are required for gamete fusion
Abstract
To trigger gamete fusion, spermatozoa need to activate the molecular machinery in which sperm IZUMO1 and oocyte JUNO (IZUMO1R) interaction plays a critical role in mammals. Although a set of factors involved in this process has recently been identified, no common factor that can function in both vertebrates and invertebrates has yet been reported. Here, we first demonstrate that the evolutionarily conserved factors dendrocyte expressed seven transmembrane protein domain-containing 1 (DCST1) and dendrocyte expressed seven transmembrane protein domain-containing 2 (DCST2) are essential for sperm–egg fusion in mice, as proven by gene disruption and complementation experiments. We also found that the protein stability of another gamete fusion-related sperm factor, SPACA6, is differently regulated by DCST1/2 and IZUMO1. Thus, we suggest that spermatozoa ensure proper fertilization in mammals by integrating various molecular pathways, including an evolutionarily conserved system that has developed as a result of nearly one billion years of evolution.
Introduction
Gamete recognition and fusion in mammals are considered to occur through a complex intermolecular interaction in which izumo sperm–egg fusion 1 (IZUMO1), sperm acrosome associated 6 (SPACA6), transmembrane protein 95 (TMEM95), fertilization influencing membrane protein (FIMP) and sperm–oocyte fusion required 1 (SOF1) on the sperm side and JUNO (also known as IZUMO1 receptor) and cluster of differentiation 9 (CD9) on the ovum side are all involved in membrane fusion, as proven by gene disruption (Barbaux et al., 2020; Bianchi et al., 2014; Fujihara et al., 2020; Inoue et al., 2005; Kaji et al., 2000; Lamas-Toranzo et al., 2020; Le Naour et al., 2000; Lorenzetti et al., 2014; Miyado et al., 2000; Noda et al., 2020). Particularly, the IZUMO1–JUNO interaction is likely to be essential for triggering gamete fusion (Aydin et al., 2016; Inoue et al., 2015; Inoue et al., 2013; Ohto et al., 2016); however, it is unknown how these factors contribute to this process (Bianchi and Wright, 2020).
Here, we show that an osteoclast fusion-related factor, dendrocyte expressed seven transmembrane protein (DC-STAMP) (Kukita et al., 2004; Yagi et al., 2005), homologue DC-STAMP domain-containing 1 (DCST1), and its paralogue DCST2, which show testis and haploid-specific expression (Figure 1D), are indispensable for fertilization in mice. Mouse DCST1 is considered to be an orthologue of Caenorhabditis elegans spermatogenesis-defective 49 (SPE-49) (Wilson et al., 2018) and Drosophila SNEAKY (Wilson et al., 2006), whereas mouse DCST2 is considered to be an orthologue of C. elegans SPE-42 (Kroft et al., 2005) and Drosophila DCST2, although the numbers of putative transmembrane regions appear different (Figure 1A,B). Previously, these factors have been shown to be essential for fertilization. In fact, phylogeny analysis revealed that DCST1 and DCST2 belong to different clades (Figure 1C). This supports the finding that DCST2 appears to be closer to ancestor molecules than DCST1, because DCST1 is considered to have arisen after branching from DCST2 (Figure 1C).
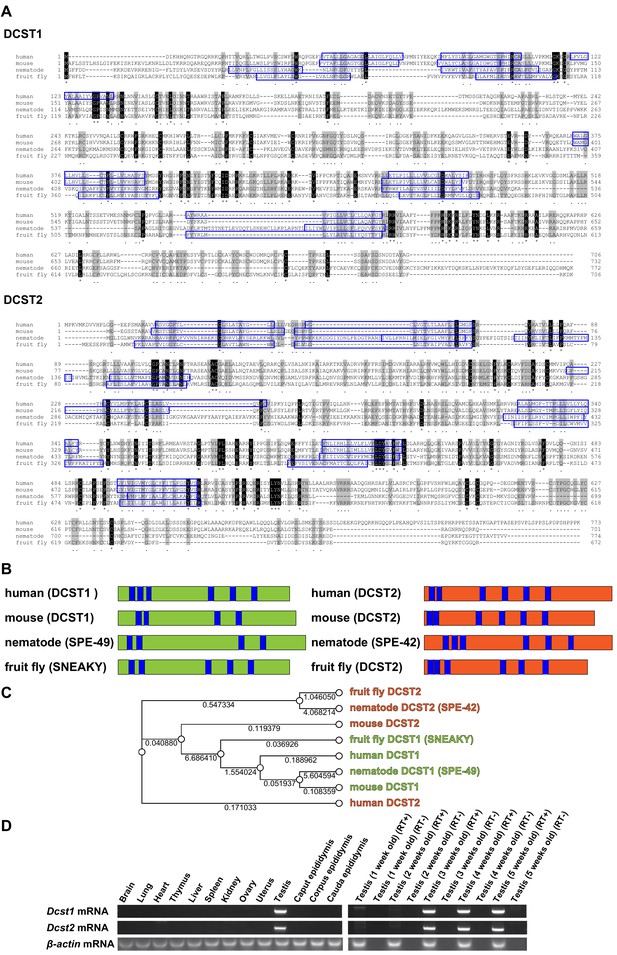
Alignments of dendrocyte expressed seven transmembrane protein domain-containing 1 (DCST1) and dendrocyte expressed seven transmembrane protein domain-containing 2 (DCST2) amino acid sequences, and mRNA expression profiles.
(A) Sequence alignments of DCST1 in Homo sapiens (human; GenBank: NP_689707.2), Mus musculus (mouse; GenBank: NP_084250.1), Caenorhabditis elegans (nematode; GenBank: NP_001343858.1), and Drosophila guanche (fruit fly; GenBank: XP_034122086.1) are shown. Sequence alignments of DCST2 in Homo sapiens (human; GenBank: NP_653223.2), Mus musculus (mouse; GenBank: NP_001357782.1), Caenorhabditis elegans (nematode; GenBank: NP_872213.4), and Drosophila mauritiana (fruit fly; GenBank: XP_033160419.1) are shown. Identical and similar amino acid residues are represented as asterisks and dots, respectively. The residues of putative transmembrane domains are indicated by blue boxes. (B) Schematic diagram of DCST1 and DCST2 in various species. The blue area indicates the putative transmembrane domain. (C) Phylogeny analysis of DCST1 and DCST2. Maximum likelihood phylogenetic tree was constructed using RAxML with 1000 bootstraps through Genetyx software. The distance is shown as a numerical value. (D) Tissue and age-dependent expression profile of Dcst1 and Dcst2 mRNA in mice. β-actin mRNA was used as an internal control.
For the first time, we here provide evidence that a common set of related factors between vertebrates and invertebrates actively participates in fertilization and appears to promote gamete merging in mammals.
Results and discussion
Since Dcst1 and Dcst2 are transcribed in different directions so as to face each other (Figure 2A), we first produced Dcst1/2 doubly-disrupted mouse lines using the homologous recombination system in embryonic stem cells, replacing Dcst1 exon 1–3 and Dcst2 exon 1–4, including the promoter region with the neomycin-resistance gene (Neor) (Figure 2A,B). The Dcst1/2-/- male mice, but not female, became completely infertile (Figure 3E). When eggs were collected from the oviducts of the female mice 8 hr after coitus with a Dcst1/2-/- male, many zona-penetrated spermatozoa that had not been fertilized, and possessed a normal IZUMO1 localization upon acrosome reaction, were seen in the perivitelline space of oocytes (Figure 3A). There was no difference in sperm motility between the Dcst1/2-/- and wild-type mice (motility was measured 120 min after incubation by computer-assisted sperm motility analysis in CEROS; mean ± s.e.m = 97.0 ± 0.73% in wild-type [1522 spermatozoa, six individuals] and 96.8 ± 0.87% in Dcst1/2-/- [1425 spermatozoa, six individuals]). Therefore, it is reasonable to assume that there are no disturbances in sperm migration into the oviduct, acrosome reaction, and zona penetration (Figure 3A). More precisely, gamete fusion assay showed that Dcst1/2-/- spermatozoa were capable of binding to the plasma membranes of oocytes, but no Dcst1/2-/- spermatozoa had fused with the oocytes (Figure 3B). Since the syngamy was restored by bypassing with intracytoplasmic sperm injection (ICSI) using Dcst1/2-/- spermatozoa (numbers of pups born from Dcst1/2+/- and Dcst1/2-/- spermatozoa per survived transplanted 2 cell embryos were 8/27 [30%] and 17/48 [35%], respectively), DCST1/2 should be an essential part of gamete fusion machinery. Furthermore, the oocyte fusion-related factors JUNO and CD9 were found to be concentrated in regions, at which acrosome-reacted (AR) spermatozoa from Dcst1/2-deficient mice labeled with α-IZUMO1 antibody were bound, same as wild-type spermatozoa (Figure 3C, Inoue et al., 2020), suggesting that Dcst1/2-/- spermatozoa are capable of recruiting the oocyte factors at the contact site; however, these spermatozoa fail to proceed to membrane fusion with oocytes.
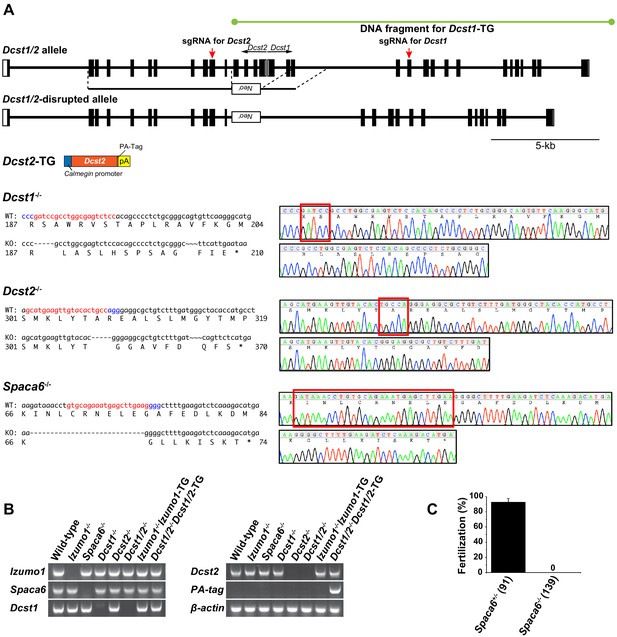
Strategy for Dcst1 and Dcst2 genes disruption and its validation.
(A) Targeted disruption of the Dcst1/2, Dcst1, Dcst2, and Spaca6 genes, and constructs for Dcst1 and Dcst2 transgenic mice. Complete structures of the wild-type mouse Dcst1/2 alleles are shown. Exons and introns are represented by boxes and horizontal lines, respectively. An open reading frame for Dcst1 and Dcst2 genes is shown in black. For the targeted disruption of mouse Dcst1/2, the neomycin-resistance gene (Neor) was inserted between Dcst1 exon 3 and Dcst2 exon 4. A herpes simplex virus thymidine kinase gene was introduced into the targeting construct for negative selection. For the gene complementation experiments, transgenic mouse lines expressing dendrocyte expressed seven transmembrane protein domain-containing 1 (DCST1), in which full-length genome DNA fragment including all promoter region, exons, and introns was incorporated, and dendrocyte expressed seven transmembrane protein domain-containing 2 (DCST2), in which full-length cDNA with a PA-tag sequence was inserted between a testis-specific (pachytene spermatocyte to spermatid stage) Calmegin promoter and rabbit β-globin polyadenylation signal, were produced. For genome-editing of Dcst1, Dcst2, and Spaca6 using the CRISPR/Cas9 system, the red and blue letters show the target and protospacer adjacent motif (PAM) sequence, respectively (left panels). A DNA sequence chromatogram shows that Dcst1-/-, Dcst2-/-, and Spaca6-/- had 5-base, 5-base, and 28-base deletions, respectively. The deleted sequences are boxed in red (right panels). (B) Validation of gene disruption by reverse transcription polymerase chain reaction (RT-PCR). All primer sets employed wild-type mRNA-specific oligonucleotide sequences except for PA-tag. As a result, all gene disruptions and transgenes were confirmed appropriately. β-actin mRNA was used as an internal control. (C) In vitro fertilization analysis using Spaca6+/- and Spaca6-/- spermatozoa (n = 4 and 4, respectively). The fertilization rate was evaluated at the two-cell stage embryo. The numbers in parentheses indicate the numbers of oocytes used. The error bars represent standard error of the mean (s.e.m.).
-
Figure 2—source data 1
IVF in SPACA6 heterozygous and homozygous KO spermatozoa.
- https://cdn.elifesciences.org/articles/66313/elife-66313-fig2-data1-v1.xlsx
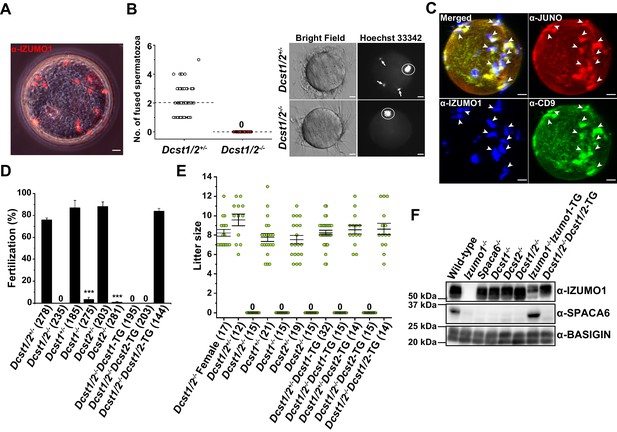
Identification of new gamete fusion-related factors dendrocyte expressed seven transmembrane protein domain-containing 1 (DCST1) and dendrocyte expressed seven transmembrane protein domain-containing 2 (DCST2).
(A) Accumulation of many acrosome-reacted (AR) IZUMO1 positive spermatozoa (red) in the perivitelline space of eggs recovered from the oviducts of females 8 hr after coitus with a Dcst1/2-/- male. Scale bar 10 µm. (B) Gamete fusion assay of Dcst1/2-disrupted spermatozoa. Gamete fusion was visualized with Hoechst 33342 dye transfer. The numbers of fused spermatozoa per oocyte from four independent experiments are shown. The total numbers of oocytes examined in Dcst1/2+/- and Dcst1/2-/- were 77 and 80, respectively (left graph). The broken lines indicate the average. The arrows and the circles in the representative photo show fused spermatozoa and meiosis metaphase II chromosomes, respectively (right picture). Scale bar 10 µm. (C) Localization of oocyte CD9 and JUNO upon Dcst1/2-/- sperm attachment. Sperm–oocyte interaction was visualized by staining with 0.25 µg ml−1 TH6–Alexa647 (JUNO: red), 0.5 µg ml−1 MZ3–FITC (CD9: green) and 0.5 µg ml−1 Mab125–Alexa546 (IZUMO1: blue). The eggs were fixed 30 min after insemination of Dcst1/2-/- spermatozoa. The arrowheads indicate the sites where JUNO and cluster of differentiation 9 (CD9) were concentrated in response to the AR spermatozoa (IZUMO1 positive: blue) bound to the oolemma. Scale bar 10 µm. (D) In vitro fertilization assay using Dcst1/2+/-, Dcst1/2-/-, Dcst1+/-, Dcst1-/-, Dcst2+/-, Dcst2-/-, Dcst1/2-/-Dcst1-TG, Dcst1/2-/-Dcst2-TG, and Dcst1/2-/-Dcst1/2-TG spermatozoa (n = 5, 5, 6, 7, 8, 8, 6, 6, and 6, respectively). The fertilization rate was evaluated at the two-cell stage embryo. The numbers in parentheses indicate the numbers of oocytes used. The error bars represent s.e.m. ***p<0.001 (paired two-tailed Student’s t-test, compared with each heterozygous mutants). (E) Fecundity of DCST1/2-related deficient male and female mice. The numbers in parentheses indicate the numbers of mating pairs. Regarding Dcst1/2-/-, Dcst1-/-, Dcst2-/-, Dcst1/2-/-Dcst1-TG, and Dcst1/2-/-Dcst2-TG males, the numbers of vaginal plug formations were counted. The error bars represent s.e.m. (F) Western blot analysis of various types of mouse lines with 1 µg ml−1 Mab18 (IZUMO1) and 1:500 SPACA6 anti-serum. Each lane was equally applied with 20 µg sperm lysates. BASIGIN was used as a loading control.
-
Figure 3—source data 1
Gamete fusion assay, IVF and litter size in DCST1/2-related mutants.
- https://cdn.elifesciences.org/articles/66313/elife-66313-fig3-data1-v1.xlsx
In order to know which factor plays a more crucial role in fertilization, we next produced solo Dcst1-/- and Dcst2-/- mouse lines using CRISPR/Cas9-mediated gene disruption, and assessed male fertilization ability simultaneously with performed transgenic rescued experiments (Figure 2A,B). In in vitro fertilization (IVF), as expected, all of the Dcst1/2-/-, Dcst1-/- and Dcst2-/- spermatozoa failed to fertilize the eggs, where the average fertilization rates of Dcst1/2-/-, Dcst12-/-, Dcst2-/- spermatozoa were 0%, 3.3%, and 0.8%, respectively (Figure 3D). The impaired fertilization step undoubtedly followed zona penetration, because motile Dcst1/2-deficient spermatozoa penetrated the zona pellucida and accumulated in the perivitelline space of the oocytes (Video 1). It is noteworthy that the spermatozoa from the single gene transgenic rescued mice (Dcst1-TG or Dcst2-TG) with Dcst1/2 knockout genetic backgrounds were unsuccessful in fertilization; however, the spermatozoa from the double transgenic mice (Dcst1/2-/-Dcst1/2-TG) had normal fertility compared to that of the Dcst1/2+/- mice (Figure 3D). Regarding the numbers of offspring, which is the final outcome of fertility, all Dcst1/2-/-, Dcst1-/-, and Dcst2-/- males were completely sterile despite normal mating behavior with ejaculation and vaginal plug formation, whereas the double transgenic rescued males (Dcst1/2-/-Dcst1/2-TG) showed normal litter sizes, consistent with IVF (Figure 3D,E). From these results, we conclude that both DCST1 and DCST2 are required for establishing the gamete fusion leading to embryogenesis.
6 hr after insemination of Dcst1/2-disrupted spermatozoa.
The first and second half of the video show Dcst1/2 heterozygous and homozygous KO spermatozoa insemination, respectively.
Finally, we investigated a protein profile of sperm factors such as IZUMO1 and SPACA6, which are essential factors for gamete recognition and fusion (Figure 2A,C; Barbaux et al., 2020; Inoue et al., 2005; Lorenzetti et al., 2014; Noda et al., 2020). As a result, in Izumo1, Spaca6, Dcst1/2, Dcst1, and Dcst2-deficient spermatozoa, SPACA6 typically disappears from the mature spermatozoa, whereas the IZUMO1 protein remains, except in Izumo1-null spermatozoa (Figure 3F). However, loss of SPACA6 was recovered by Izumo1 complementation (Izumo1-/-Izumo1-TG) (Figure 3F). Thus, IZUMO1 and SPACA6 are likely to be cooperative factors. Unexpectedly, although Dcst1/2-deficient male fertility could be restored in the Dcst1/2 transgenic rescued mice (Figure 3D,E), retrieval of SPACA6 was not observed (Figure 3F). While we do not have the data to explain this, the result implies that SPACA6 seems to be dispensable for fertilization in Dcst1/2-/-Dcst1/2-TG spermatozoa. In this context, it is interesting that DCST1 has a ubiquitin ligase activity (Nair et al., 2016). It is conceivable that precise temporal expression of putative substrates negatively regulated by ubiquitin-mediated degradation by DCST1/2 during spermatogenesis has to be required for SPACA6 protein stability, which could have been impaired in the Dcst1/2 complementation.
It should be emphasized that DCST1/2 are the first identified well-conserved factors from human to nematode or fruit fly that are actively involved in gamete recognition and fusion. Although the detailed molecular mechanisms underlining gamete recognition and fusion are still unknown, new insight into molecular behavior and interaction through DCST1 and DCST2 involvement would greatly advance our understanding of elaborate common molecular mechanisms in mysterious fertilization in a sexually reproducing organism.
Materials and methods
Reagent type (species) or resource | Designation | Source or reference | Identifiers | Additional information |
---|---|---|---|---|
Strain, strain background (Mus musculus) | Dcst1/2 KO | This article | Deposited in RIKEN BRC (ID RBRC05733) | |
Strain, strain background (Mus musculus) | Dcst1 KO | This article | Deposited in RIKEN BRC (ID RBRC10275) | |
Strain, strain background (Mus musculus) | Dcst2 KO | This article | Deposited in RIKEN BRC (ID RBRC10366) | |
Strain, strain background (Mus musculus) | Spaca6 KO | This article | Deposited in RIKEN BRC (ID RBRC10367) | |
Strain, strain background (Mus musculus) | Izumo1 KO | Inoue et al., 2005 | RRID MGI_3576518 | |
Strain, strain background (Mus musculus) | Dcst1 TG | This article | Deposited in RIKEN BRC (ID RBRC05856) | |
Strain, strain background (Mus musculus) | Dcst2 TG | This article | ||
Strain, strain background (Mus musculus) | Izumo1 TG | Inoue et al., 2005 | ||
Antibody | Anti-mouse SPACA6 (rabbit polyclonal) | This article | (WB: 1:500) | |
Antibody | Anti-mouse IZUMO1 (rat monoclonal) | Inoue et al., 2013 | Mab125 | Gift from Dr. Ikawa (IF: 0.5 µg ml−1) |
Antibody | Anti-mouse IZUMO1 (rat monoclonal) | Inoue et al., 2015 | Mab18 | (WB: 1 µg ml−1) |
Antibody | Anti-mouse FR4 (JUNO) (rat monoclonal) | BioLegend | TH6, RRID AB_1027724 | (IF: 0.25 µg ml−1) |
Antibody | Anti-mouse CD9 (rat monoclonal) | BioLegend | MZ3, RRID AB_1279321 | (IF: 0.5 µg ml−1) |
Antibody | Anti-mouse EMMPRIN (BASIGIN) (goat polyclonal) | Santa Cruz Biotechnology | G-19, RRID AB_2066959 | (WB: 0.2 µg ml−1) |
Recombinant DNA reagent | Plasmid pX330 vector | Addgene | RRID Addgene_42230 | |
Sequence-based reagent | Primers | This article | Detailed in 'Materials and methods' | |
Peptide, recombinant protein | SPACA646-139 | This article | Detailed in 'Materials and methods' |
Mice
C57BL/6, B6D2F1, and ICR mice (Mus musculus) were purchased from Japan SLC Inc, and IZUMO1 knockout and transgenic mice (Inoue et al., 2005) were kindly provided by Osaka University. All animal studies were approved by the Animal Care and Use Committee of Fukushima Medical University, Japan, and performed under the guidelines and regulations of Fukushima Medical University.
Antibodies
An α-mouse IZUMO1 monoclonal antibody (Mab125) was conjugated to Alexa Fluor 546. FITC–conjugated α-mouse CD9 monoclonal (MZ3) and Alexa Fluor 647–labeled α-mouse JUNO monoclonal (TH6) antibodies were purchased from BioLegend. Unlabeled α-mouse BASIGIN polyclonal antibody (sc-9757) was purchased from Santa Cruz Biotechnology.
Generation of Dcst1/2 double knockout mice
Request a detailed protocolA targeting vector was constructed using modified pNT1.1 containing neomycin-resistance gene as a positive selection marker and herpes simplex virus thymidine kinase gene as a negative selection marker (http://www.ncbi.nlm.nih.gov/nuccore/JN935771). A 1.7 kb Not I-Xho I fragment and a 6.8 kb Pac I-Mfe I fragment were inserted as short and long arms, respectively. Embryonic stem cells derived from 129/Sv were electroporated with Cla I digested linearized DNA. Of the 192 G418-resistant clones, five had undergone homologous recombination correctly. Two targeted cell lines were injected into C57BL/6 mice derived blastocysts, resulting in the birth of male chimeric mice. These mice were then crossed with C57BL/6 to obtain heterozygous mutants. The mice used in the present study were the offspring of crosses between F1 and/or F2 generations. The PCR primers used for genotyping were as follows: 5'-ATTCTTCCTCTCCCTTTCGGACATC-3' and 5'- GCTTGCCGAATATCATGGTGGAAAATGGCC-3' for the short arm side of the mutant allele; 5'-ATTCTTCCTCTCCCTTTCGGACATC-3' and 5'-CCAGGGGATCCAACACCCTT-3' for the short arm side of the wild-type allele; 5'-TCTGTTGTGCCCAGTCATAGCCGAATAGCC-3' and 5'-CTGGAAGTGTCTTCCGAGTGCCACTCCACA-3' for the long arm side of the mutant allele; and 5'-CCCTAGGCTGTGAAGTGTTGATGAG-3' and 5'-CTGGAAGTGTCTTCCGAGTGCCACTCCACA-3' for the long arm side of the wild-type allele. The Dcst1/2-disrupted mouse line was submitted to RIKEN BioResource Research Center (https://web.brc.riken.jp/en/), and is available to the scientific community (accession number: RBRC05733).
Generation of Dcst1-/-, Dcst2-/-, and Spaca6-/- mice
Request a detailed protocolPairs of oligonucleotides (5’-CACCGGGAGACTCGCCAGGCGGATC-3’ and 5’-AAACGATCCGCCTGGCGAGTCTCCC-3’ for DCST1, 5’-CACCGGCATGAAGTTGTACACTGCC-3’ and 5’-AAACGGCAGTGTACAACTTCATGCC-3’ for DCST2, and 5’-CACCGGTGCAGAAATGAGCTTGAAG-3’ and 5’-AAACCTTCAAGCTCATTTCTGCACC-3’ for SPACA6) as guide sequences were annealed and cloned into a Bbs I cut pX330 vector (Addgene plasmid #42230). pX330 plasmid DNA was purified and diluted into 5 mM Tris-HCl pH 7.4/0.1 mM EDTA of a final 5 ng μl−1 concentration (Mashiko et al., 2013). Plasmid DNA was injected into the pronuclei of fertilized eggs with glass capillaries attached to a micromanipulator (FemtoJet, Eppendorf). After microinjection, the zygotes were transferred and cultured in KSOM (ark-resource) at 37°C with 5% CO2, and two-cell embryos were transferred into pseudopregnant ICR female mice. F3 and F4 generation mice were used for the experiments, and all genotyping was performed by PCR on tail-tip DNA using: DCST1, 5’-CATCAGCCAGTAGCCCCAAA-3’ and 5’-AGATCAACAACACCCGATCC-3’ for wild-type or 5’-CAGATCAACAACACCCGCCT-3’ for KO as forward and reverse primers, respectively, and DCST2, 5’-TAGAGAAAGGCAGAAAGTCCAGAC-3’ and 5’-AAGACAGCGCCTCCCTGGCA-3’ for wild-type or 5’-AAAGACAGCGCCTCCCGTGT-3’ for KO and as forward and reverse primers, respectively. SPACA6, 5’-TTTTCTGTCTCTGTCTCTTATGCCAA-3’ and 5’- TTCAAAAGCCCCTTCAAGCTCA-3’ for wild-type or 5’-AGATCTTCAAAAGCCCCTTGGTTT-3’ were used for KO and as forward and reverse primers, respectively. DCST1, DCST2, and SPACA6 knockout mice were deposited at the RIKEN BioResource Research Center (https://web.brc.riken.jp/en/) (accession numbers RBRC10275, RBRC10366 and RBRC10367, respectively).
Production of DCST1 and DCST2 transgenic mice
Request a detailed protocolDcst1 gene fragments were amplified by PCR using a bacterial artificial chromosome (BAC) DNA clone (RP24-185C5) as template DNA. The DNA fragments were ligated into StrataClone PCR Vector pSC-B-amp/kan (Agilent Technologies). Until injection, a 17.5 kb DNA fragment that included whole Dcst1 gene was digested with Not I and Sal I (Figure 2A). For DCST2, we designed PA-tagged Dsct2, to be inserted between the Calmegin promoter (testis specific promoter) and a rabbit β-globin polyadenylation signal (Figure 2A). The transgenic mouse lines were produced by injecting Dcst1 gene or Dsct2-PA DNA fragments into the pronuclei of fertilized eggs. For the detection of transgene, PCR was performed on tail-tip DNA using following primer sets: 5’-GGCCGCTCTAGAACTAGTGGAT-3’ and 5’-GGTGTTTGTGTTACTGAAGTCACTG-3’ for DCST1-TG specific amplification, and 5’-CCTTCCTGCGGCTTGTTCTCT-3’ and 5’-GGCTATGTGTTTCACGGCAT-3’ for DCST2-TG specific amplification.
RT-PCR
Request a detailed protocolTotal RNA was extracted from a mouse testis using NucleoSpin RNA Plus and treated with rDNase (MACHEREY-NAGEL). First-strand cDNA synthesis was performed using a PrimeScriptII 1 st strand cDNA Synthesis Kit with Oligo (dT)15 primer (Takara Bio). The following primer pairs were designed to amplify each mRNA: IZUMO1 wild-type specific primer pair, forward (5’-CCTATTTGGGGGCCGTGGAC-3’) and reverse (5’-GTCCAGGACCAGGTCTTCCGAGCGA-3’); SPACA6 wild-type specific primer pair, forward (5’-ACCCGGAGACCCTCTGTTAT-3’) and reverse (5’-TTCAAAAGCCCCTTCAAGCTCA-3’); DCST1 wild-type specific primer pair, forward (5’-CTCATCAACACTTCACAGCCTAGGG-3’) and reverse (5’-GGAGACTCGCCAGGCGGATC-3’); DCST2 wild-type specific primer pair, forward (5’-ATGCCGTGAAACACATAGCC-3’) and reverse (5’-AAGACAGCGCCTCCCTGGCA-3’); PA-tag specific primer pair, forward (5’-ATGGAGAACTTTGTGTCCTGCAGTA-3’) and reverse (5’-ACCACATCATCTTCGGCACCTGGCAT-3’); β-actin specific primer pair, forward (5’-TGACAGGATGCAGAAGGAGA-3’) and reverse (5’-GCTGGAAGGTGGACAGTGAG-3’) primer set (Saito et al., 2019). PCR was carried out using TaKaRa Ex Taq Hot Start Version (Takara bio).
Gamete fusion assay
View detailed protocolSuperovulation was induced in each B6D2F1 female mouse (>8 weeks old) by injecting 7.5 IU of equine chorionic gonadotropin (eCG) and 7.5 IU of human chorionic gonadotropin (hCG) at 48 hr intervals. Oocytes were collected from the oviduct 16 hr after hCG injection, and placed in 50 μl of Toyoda, Yokoyama and Hoshi (TYH) medium. The zona pellucida was removed from the oocytes via treatment with 1.0 mg ml−1 of collagenase (FUJIFILM Wako Pure Chemical Corporation) (Yamatoya et al., 2011). They were preloaded with 1 µg ml−1 Hoechst 33342 (Thermo Fisher Scientific) in TYH medium for 10 min, and were washed prior to the addition of the spermatozoon. Dcst1/2-/- spermatozoa were collected from the cauda epididymis, and capacitated in vitro for 2 hr in a 200 µl drop of TYH medium. Zona-free oocytes were co-incubated with 2 × 105 mouse spermatozoa ml−1 for 30 min at 37°C in 5% CO2. After 30 min of incubation, the eggs were observed under a fluorescence microscope after being fixed with 0.25% glutaraldehyde in flushing holding medium (FHM) medium. This procedure enabled staining of fused sperm nuclei only, by transferring the dye into spermatozoa after membrane fusion.
Fluorescence imaging
Request a detailed protocolZona-free oocytes were prepared as described above. They were incubated with 0.5 µg ml−1 MZ–FITC and 0.25 µg ml−1 TH6–Alexa647 for 1 hr at 37°C in TYH medium. Dcst1/2-/- spermatozoa were collected from the cauda epididymis, and capacitated in vitro for 2 hr in a 200 µl drop of TYH medium with 0.5 µg ml−1 Mab125–Alexa546 that was covered with mineral oil (Merck). Then, 2 × 105 spermatozoa ml−1 was inseminated in the oocytes for 30 min at 37°C with 5% CO2. After the eggs were washed three times in TYH medium by transferring spots of oocytes containing media, the eggs were fixed with 4% paraformaldehyde and 0.5% polyvinylpyrrolidone (PVP) in phosphate buffered saline (PBS) for 30 min at room temperature, then observed in FHM medium on the glass-bottom dishes (No. 0, MatTek). A 100× oil-immersion objective (numeric aperture 1.49) was used to capture confocal images with an A1R microscope (Nikon). The pinhole was set at 3.0 airy unit. For 3D reconstruction, 100–120 fluorescent images were taken at 1 μm intervals on the Z axis, and then 3D images were reconstructed using the built-in software NIS-Elements ver. 4.3 (Nikon).
In vitro fertilization
Request a detailed protocolMouse spermatozoa were collected from the cauda epididymis, and were capacitated in vitro for 2 hr in a 200 µl drop of TYH medium that was covered with mineral oil. Superovulation was induced in female mouse, as described above. Oocytes were collected from the oviduct placed in 100 μl of TYH medium, and incubated with 2 × 105 spermatozoa ml−1 at 37°C with 5% CO2. After 5 hr of insemination, the oocytes were washed with TYH medium and the fertilization rate was evaluated at the two-cell stage embryo.
Production of SPACA6 polyclonal antibody
Request a detailed protocolDNA coding for the extracellular region of mouse SPACA646-139 was constructed using synthetic DNA with codon use that was optimized for expression in Escherichia coli. The SPACA646-139 fragment was amplified by PCR with a 3’-primer with sequence encoding Ala-Gly-Gly-His-His-His-His-His-His, a linker plus a hexahistidine tag. The amplified PCR products were cloned back into pAED4 (Doering and Matsudaira, 1996). The construct was expressed using E. coli strain BL21 (DE3) pLysS (Agilent Technologies). The expressed SPACA646-139 fragment was accumulated in inclusion body. Inclusion body from 0.8 l culture was solubilized by the addition of 5 g of solid guanidine hydrochloride (GdnHCl), followed by the addition of 1 ml of 1M Tris-HCl (pH 8.5). The sample was subjected to HiTrap metal-chelating column (cytiva), which was equilibrated with 6 M urea, 10 mM Tris-HCl (pH 8.5), and 0.5 M NaCl, and eluted using imidazole gradient. Then, the sample was dialyzed against 10 mM Tris-HCl (pH 8.5) for a few days, filtered using a 0.45 µm filter (Merck Millipore), and concentrated by Amicon Ultra 10 (Merck Millipore). Protein stock concentration was determined by measuring the optical density at 280 nm based on the absorption of Trp and Tyr residues using the Edelhoch spectral parameters (Gill and von Hippel, 1989).
The SPACA646-139 fragment was mixed and emulsified with Freund’s complete or incomplete adjuvant (BD Difco) and used for immunization of rabbits. Immunization was performed four times at 7-day intervals, and booster immunizations were administered before drawing blood. After purifying the anti-serum, antibody was kept at −80°C until use.
Western blot analysis
Request a detailed protocolMouse spermatozoa were collected from the cauda epididymis and were solubilized with 1% Triton X-100 and PBS with a protease inhibitor cocktail (nacalai tesque). Samples were treated with SDS-sample buffer (62.5 mM Tris-HCl [pH 6.8], 2% SDS, 50 mM dithiothreitol, 10% glycerol), then used for western blotting under reduced conditions. The membranes were probed with primary antibodies (1 µg ml−1 α-IZUMO1 [Mab18], 1:500 α-SPACA6, and 0.2 µg ml−1 α-BASIGIN [sc-9757]) followed by secondary antibodies conjugated to horseradish peroxidase (HRP) (Jackson ImmunoResearch Laboratories). Chemiluminescence reactions were performed with ECL Prime (cytiva).
Statistical analyses
Request a detailed protocolAll statistical analyses were performed using OriginPro 2020b (Light Stone).
Data availability
All data generated or analysed during this study are included in the manuscript.
References
-
Calculation of protein extinction coefficients from amino acid sequence dataAnalytical Biochemistry 182:319–326.https://doi.org/10.1016/0003-2697(89)90602-7
-
The gamete fusion process is defective in eggs of Cd9-deficient miceNature Genetics 24:279–282.https://doi.org/10.1038/73502
-
RANKL-induced DC-STAMP is essential for osteoclastogenesisJournal of Experimental Medicine 200:941–946.https://doi.org/10.1084/jem.20040518
-
The Caenorhabditis elegans spe-49 gene is required for fertilization and encodes a sperm-specific transmembrane protein homologous to SPE-42Molecular Reproduction and Development 85:563–578.https://doi.org/10.1002/mrd.22992
-
DC-STAMP is essential for cell–cell fusion in osteoclasts and foreign body giant cellsJournal of Experimental Medicine 202:345–351.https://doi.org/10.1084/jem.20050645
-
One-step collagenase method for zona pellucida removal in unfertilized eggs: easy and gentle method for large-scale preparationReproductive Medicine and Biology 10:97–103.https://doi.org/10.1007/s12522-011-0075-8
Article and author information
Author details
Funding
Japan Society for the Promotion of Science (JP18H02453)
- Naokazu Inoue
Japan Society for the Promotion of Science (JP17K07311)
- Ikuo Wada
Takeda Science Foundation
- Naokazu Inoue
Gunma University (19014)
- Naokazu Inoue
The funders had no role in study design, data collection and interpretation, or the decision to submit the work for publication.
Acknowledgements
We thank Dr. Kazuo Yamagata at Kindai University and the Biotechnology Research and Development (non-profit organization) at Osaka University for their technical assistance in ICSI and making Dcst1/2 knockout mice, respectively.
Ethics
Animal experimentation: All animal studies were approved by the Animal Care and Use Committee of Fukushima Medical University, Japan (approval number: 2020017), and performed under the guidelines and regulations of Fukushima Medical University.
Copyright
© 2021, Inoue et al.
This article is distributed under the terms of the Creative Commons Attribution License, which permits unrestricted use and redistribution provided that the original author and source are credited.
Metrics
-
- 3,954
- views
-
- 309
- downloads
-
- 64
- citations
Views, downloads and citations are aggregated across all versions of this paper published by eLife.
Download links
Downloads (link to download the article as PDF)
Open citations (links to open the citations from this article in various online reference manager services)
Cite this article (links to download the citations from this article in formats compatible with various reference manager tools)
Further reading
-
- Developmental Biology
Apical constriction is a basic mechanism for epithelial morphogenesis, making columnar cells into wedge shape and bending a flat cell sheet. It has long been thought that an apically localized myosin generates a contractile force and drives the cell deformation. However, when we tested the increased apical surface contractility in a cellular Potts model simulation, the constriction increased pressure inside the cell and pushed its lateral surface outward, making the cells adopt a drop shape instead of the expected wedge shape. To keep the lateral surface straight, we considered an alternative model in which the cell shape was determined by cell membrane elasticity and endocytosis, and the increased pressure is balanced among the cells. The cellular Potts model simulation succeeded in reproducing the apical constriction, and it also suggested that a too strong apical surface tension might prevent the tissue invagination.
-
- Cancer Biology
- Developmental Biology
Missense ‘hotspot’ mutations localized in six p53 codons account for 20% of TP53 mutations in human cancers. Hotspot p53 mutants have lost the tumor suppressive functions of the wildtype protein, but whether and how they may gain additional functions promoting tumorigenesis remain controversial. Here, we generated Trp53Y217C, a mouse model of the human hotspot mutant TP53Y220C. DNA damage responses were lost in Trp53Y217C/Y217C (Trp53YC/YC) cells, and Trp53YC/YC fibroblasts exhibited increased chromosome instability compared to Trp53-/- cells. Furthermore, Trp53YC/YC male mice died earlier than Trp53-/- males, with more aggressive thymic lymphomas. This correlated with an increased expression of inflammation-related genes in Trp53YC/YC thymic cells compared to Trp53-/- cells. Surprisingly, we recovered only one Trp53YC/YC female for 22 Trp53YC/YC males at weaning, a skewed distribution explained by a high frequency of Trp53YC/YC female embryos with exencephaly and the death of most Trp53YC/YC female neonates. Strikingly, however, when we treated pregnant females with the anti-inflammatory drug supformin (LCC-12), we observed a fivefold increase in the proportion of viable Trp53YC/YC weaned females in their progeny. Together, these data suggest that the p53Y217C mutation not only abrogates wildtype p53 functions but also promotes inflammation, with oncogenic effects in males and teratogenic effects in females.