Chaperones: Aggregation in the spotlight
From bacteria to plants to humans, all organisms carry a group of molecular chaperones dedicated to helping cells withstand various stressors such as heat, drought, aging or injury. These small heat shock proteins (or sHsps) help cells adapt to stress by associating with damaged proteins. Without this help, the proteins may form insoluble, harmful aggregates such as amyloid fibrils, which are associated with aging and disease.
Yet, seemingly conflicting evidence has made it difficult to understand how sHsps work. Traditionally, they are thought to prevent proteins from aggregating; however, in some cases sHsps seem to actually promote protein accumulation (Haslbeck and Vierling, 2015; Escusa-Toret et al., 2013; Mymrikov et al., 2017; Walther et al., 2015). Intuitively inconsistent, this picture suggests that the sHsp mechanism is yet to be fully pieced together (Mogk et al., 2019). Now, in eLife, Florian Stengel and colleagues from institutions in Germany and Italy – including Edgar Boczek and Julius Fürsch as first co-authors – report how this apparent contradiction might be reconciled (Boczek et al., 2021).
The team built on observations from 35 years ago that sHsps are found in membrane-free structures that often form in cells under stress (Collier and Schlesinger, 1986). Now understood to be droplet-like, these ‘condensates’ store select proteins and other biological molecules, keeping them safely huddled together until the cell recovers (Wallace et al., 2015). But proteins being present in such high concentrations can also be dangerous. If they become unstable and misfold in the condensates, there is an increased risk that they will start to form amyloid fibrils (Alberti et al., 2017; Zbinden et al., 2020). In this perilous environment, sHsps may be key to protect protein stability.
This possibility inspired Boczek et al. to study how a sHsp present in the human brain and muscles (HspB8, also known as HSP22) interacts with an RNA-binding protein that readily enters condensates and can form aggregates. Mutations within this protein – which is known as FUS, short for Fused in sarcoma – can lead to the motor neuron disease known as amyotrophic lateral sclerosis (ALS).
Boczek et al. developed a new approach that allowed them to map how the two proteins interact under different conditions. This was accomplished by combining chemical crosslinking (where compounds are used to permanently link proteins that briefly interact) and mass spectrometry (which gives a ‘snapshot’ of proteins by measuring their parts). Linking the proteins before collecting the snapshots allowed for structural insight, enabling the team to determine which parts of HspB8 and FUS were in contact inside and outside of the condensates.
This approach showed that the presence of HspB8 inside condensates containing FUS slowed down the hardening of the droplets by preventing amyloid fibrils from forming. By analysing crosslinks, Boczek et al. were able to identify which regions of the proteins are responsible for this protective mechanism. This revealed that structural changes in the segment of FUS which allows the protein to recognise RNA underlie hardening. However, when this motif is bound by a section of HspB8 known as the α-crystallin domain — an interaction that only occurs within condensates — FUS cannot turn into aggregates. In turn, a mutant version of HspB8 where the α-crystallin domain is altered was not able to protect FUS condensates against amyloid formation. This mutation in HspB8 is associated with a distal motor neuropathy, which, like amyotrophic lateral sclerosis, causes muscle weakness.
Further results showed that the disordered N-terminal domain of HspB8 was necessary for its recruitment to FUS condensates, a mechanism also observed in a related chaperone called HspB1 (Liu et al., 2020). Comparing how HspB8 and HspB1 interact with FUS helps to better understand how these chaperones work in general. In particular, unlike HspB8 which functions inside condensates, HspB1 can protect FUS both inside (if the chaperone carries a chemical modification) and outside of these structures (Liu et al., 2020). HspB8 and HspB1 are sometimes present in the same small groups of proteins, raising the question of how co-operation amongst sHsps benefits the cell (Sun et al., 2004).
A coherent picture of the sHsp mechanism is therefore gradually emerging, aided substantially by the findings of Boczek et al. It appears that, whether alone or together, sHsps can ensure that proteins do not form insoluble structures during stress. The chaperones may seem to both promote and prevent protein aggregation, but this apparent contradiction can be reconciled by considering that what researchers previously called ‘aggregation’ in fact encompasses different ways that molecules can come together. When required by the cell, sHsps help to gather proteins into condensates in which they are more densely packed than before but remain dynamic. There, the chaperones prevent proteins from forming harmful aggregates such as amyloid fibrils (Figure 1). Obtaining data on sHsp interactions in vivo across the tree of life is now needed to confirm whether this mechanism occurs in the diverse organisms that host these enigmatic chaperones.
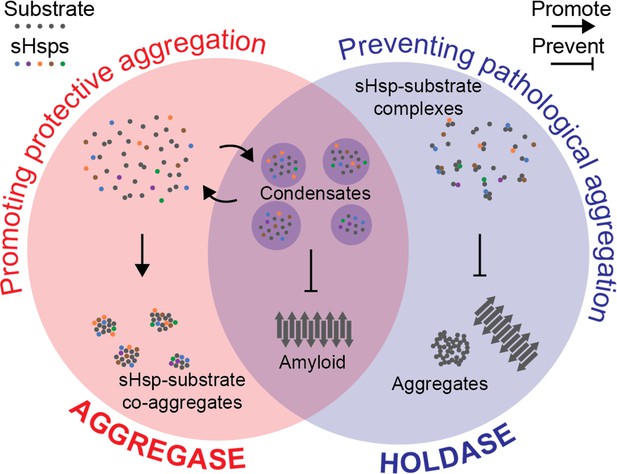
Hypothesis for a coherent mechanism of sHsp chaperone function.
Chaperones known as sHsps (colourful dots) can bind to proteins destabilised under stress conditions (‘substrates’; grey dots). They either encourage other proteins to gather with them in large protective complexes known as co-aggregates (‘aggregase’ activity; red, left); or they keep the proteins they bind apart from each other (‘holdase’ activity; blue, right) to stop these substrates from transitioning into toxic aggregates such as amyloid fibrils (grey arrows). These apparently incompatible models of chaperones’ activity can be reconciled by considering that they both aim to stop destabilised proteins from coming together in specific ways that are harmful to the cell. Sometimes, this aim is achieved by forming reversible liquid condensates requiring both aspects of sHsp function: there, the chaperones help to sequester the proteins and prevent them from becoming amyloid fibrils.
References
-
Granulostasis: Protein quality control of RNP granulesFrontiers in Molecular Neuroscience 10:84.https://doi.org/10.3389/fnmol.2017.00084
-
The dynamic state of heat shock proteins in chicken embryo fibroblastsJournal of Cell Biology 103:1495–1507.https://doi.org/10.1083/jcb.103.4.1495
-
A first line of stress defense: small heat shock proteins and their function in protein homeostasisJournal of Molecular Biology 427:1537–1548.https://doi.org/10.1016/j.jmb.2015.02.002
-
Hsp27 chaperones FUS phase separation under the modulation of stress-induced phosphorylationNature Structural & Molecular Biology 27:363–372.https://doi.org/10.1038/s41594-020-0399-3
-
Cellular functions and mechanisms of action of small heat shock proteinsAnnual Review of Microbiology 73:89–110.https://doi.org/10.1146/annurev-micro-020518-115515
-
The chaperone activity and substrate spectrum of human small heat shock proteinsJournal of Biological Chemistry 292:672–684.https://doi.org/10.1074/jbc.M116.760413
-
Interaction of human HSP22 (HSPB8) with other small heat shock proteinsJournal of Biological Chemistry 279:2394–2402.https://doi.org/10.1074/jbc.M311324200
Article and author information
Author details
Publication history
Copyright
© 2021, Wang et al.
This article is distributed under the terms of the Creative Commons Attribution License, which permits unrestricted use and redistribution provided that the original author and source are credited.
Metrics
-
- 1,490
- views
-
- 151
- downloads
-
- 1
- citations
Views, downloads and citations are aggregated across all versions of this paper published by eLife.
Download links
Downloads (link to download the article as PDF)
Open citations (links to open the citations from this article in various online reference manager services)
Cite this article (links to download the citations from this article in formats compatible with various reference manager tools)
Further reading
-
- Biochemistry and Chemical Biology
Mutations in the kinase and juxtamembrane domains of the MET Receptor Tyrosine Kinase are responsible for oncogenesis in various cancers and can drive resistance to MET-directed treatments. Determining the most effective inhibitor for each mutational profile is a major challenge for MET-driven cancer treatment in precision medicine. Here, we used a deep mutational scan (DMS) of ~5764 MET kinase domain variants to profile the growth of each mutation against a panel of 11 inhibitors that are reported to target the MET kinase domain. We validate previously identified resistance mutations, pinpoint common resistance sites across type I, type II, and type I ½ inhibitors, unveil unique resistance and sensitizing mutations for each inhibitor, and verify non-cross-resistant sensitivities for type I and type II inhibitor pairs. We augment a protein language model with biophysical and chemical features to improve the predictive performance for inhibitor-treated datasets. Together, our study demonstrates a pooled experimental pipeline for identifying resistance mutations, provides a reference dictionary for mutations that are sensitized to specific therapies, and offers insights for future drug development.
-
- Biochemistry and Chemical Biology
- Genetics and Genomics
RNA binding proteins (RBPs) containing intrinsically disordered regions (IDRs) are present in diverse molecular complexes where they function as dynamic regulators. Their characteristics promote liquid-liquid phase separation (LLPS) and the formation of membraneless organelles such as stress granules and nucleoli. IDR-RBPs are particularly relevant in the nervous system and their dysfunction is associated with neurodegenerative diseases and brain tumor development. Serpine1 mRNA-binding protein 1 (SERBP1) is a unique member of this group, being mostly disordered and lacking canonical RNA-binding domains. We defined SERBP1’s interactome, uncovered novel roles in splicing, cell division and ribosomal biogenesis, and showed its participation in pathological stress granules and Tau aggregates in Alzheimer’s brains. SERBP1 preferentially interacts with other G-quadruplex (G4) binders, implicated in different stages of gene expression, suggesting that G4 binding is a critical component of SERBP1 function in different settings. Similarly, we identified important associations between SERBP1 and PARP1/polyADP-ribosylation (PARylation). SERBP1 interacts with PARP1 and its associated factors and influences PARylation. Moreover, protein complexes in which SERBP1 participates contain mostly PARylated proteins and PAR binders. Based on these results, we propose a feedback regulatory model in which SERBP1 influences PARP1 function and PARylation, while PARylation modulates SERBP1 functions and participation in regulatory complexes.