Cytoskeleton: Capturing intermediate filament networks
Cells are highly structured systems that contain a network of filaments known as the cytoskeleton. This scaffolding structure helps cells to maintain their shape, internal organization, and integrity under stress. It also plays important roles in intracellular transport, cell signaling and in cell proliferation, growth, differentiation and death. To fulfill their purpose, cytoskeletal filaments must be spatially organized, regulated and integrated in a manner that meets the rapidly changing needs of a cell (Kim and Coulombe, 2007; Block et al., 2015).
Recent advances in microscopy and image analysis have provided transformative insight into the three-dimensional (3D) architecture of cellular components in tissues, organs and entire organisms. Now, in eLife, Rudolf Leube and colleagues at RWTH Aachen University – including Reinhard Windoffer as first author – report on new insights into networks of intermediate filaments in three different types of epithelial cells (Windoffer et al., 2022).
The researchers used confocal microscopy and newly developed image analysis tools to describe the 3D organization of the entire intermediate filament network in three types of epithelial cells: MDCK cells, which are derived from canine kidneys, HaCaT keratinocytes derived from human skin, and retinal pigment epithelial (RPE) cells from mice. To visualize the network of keratin intermediate filaments in all three cell types, a specific keratin, known as Keratin 8 (K8), was tagged with a green fluorescence marker. The 3D models were generated based on microscopy images of filaments containing fluorescent K8, and the digitized representations of these images were analyzed at different scales to quantitatively describe the properties of the filaments and the networks they form.
From a methodology standpoint alone, the study by Windoffer et al. breaks new ground and sets the stage for an atlas-type collection holding information on the organization and architecture of intermediate filaments for a plethora of cell types, under various biological conditions. Among the myriad findings reported in this article, three stand out because they help capture the breadth of the study.
First, on a cellular scale, intermediate filaments show a specific spatial organization in the three cell types analyzed. For instance, MDCK kidney cells feature distinct apical and basal keratin intermediate filament networks that are interconnected but each possess unique features (Figure 1). HaCat cells are comparatively very flat and are densely packed with keratin filaments that enclose the nucleus laterally and include long bundles that run parallel to the cell’s longest axis. Retinal pigment epithelial (RPE) cells, which were analyzed in the natural context of the eye in situ, exhibit a comparatively less dense keratin intermediate filament network that is surprisingly prominent in the cytoplasmic apical domain, which faces photoreceptor cells in the retina. The mechanisms underlying these differences, and others reported by the researchers, are certainly worth investigating.
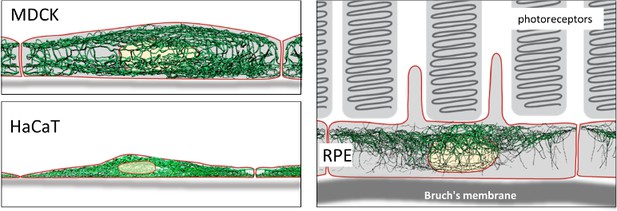
Intermediate filaments in different cell types.
Top left: Lateral view of intermediate filament networks in canine kidney epithelial cells (MDCK). The connected apical and basal networks each possess unique features. Bottom left: Human skin keratinocytes (HaCaT) are flat and densely packed, with long bundles of intermediate filaments enclosing the nucleus laterally. Right: Retinal pigment epithelial cells (RPE) in the (mouse) eye in situ show a comparatively less dense network that is surprisingly prominent in the apical area, which faces photoreceptor cells in the retina.
Image credit: Images reproduced from Windoffer et al. with permission, CC BY 4.0 (Windoffer et al., 2022).
Second, at a subcellular level, Windoffer et al. provide a thorough account of several key properties of intermediate filaments, which help inform the reciprocal interplay between mechanical forces and network architecture at both local and cell-wide levels. Again, an interesting mix of convergence and divergence are reported for the three cell types analyzed.
Third, at a molecular level, conversions of digital representations of the intermediate filaments into biochemical quantities revealed that the mass of keratin in skin keratinocytes is similar to mass measurements obtained using quantitative western blotting (Feng et al., 2013). Such detailed knowledge can help guide future efforts to identify and characterize the mechanisms that regulate the amount of keratin proteins and filaments present in several types of epithelial cells.
With this rigorous, innovative and elegant study, Windoffer et al. provide a methodological framework to probe the architecture and organizing principles of intermediate filament networks for any cell type in 3D. This contribution is timely in that it complements emerging evidence about the high-resolution structure of mature, intermediate filaments emanating from cryo-electron tomography imaging (e.g., Turgay et al., 2017; Weber et al., 2021) and also from crystallographic studies (e.g., Lee et al., 2020; Eldirany et al., 2021).
High resolution information about both core architecture of individual intermediate filaments and their spatial organization as intricate 3D networks within cells is sorely needed to foster a deeper understanding of their mechanical and non-mechanical roles in epithelial cells, and their disruption in disease. As we await additional quantitative data of intermediate filaments networks in other cell types and/or biological circumstances, the study by Windoffer et al. sets the stage for follow-up analyzes regarding the principles and mechanisms underlying the spatial organization of intermediate filaments in vivo.
References
-
Physical properties of cytoplasmic intermediate filamentsBiochimica et Biophysica Acta 1853:3053–3064.https://doi.org/10.1016/j.bbamcr.2015.05.009
-
Recent insight into intermediate filament structureCurrent Opinion in Cell Biology 68:132–143.https://doi.org/10.1016/j.ceb.2020.10.001
-
Keratin intracellular concentration revisited: implications for keratin function in surface epitheliaThe Journal of Investigative Dermatology 133:850–853.https://doi.org/10.1038/jid.2012.397
Article and author information
Author details
Publication history
Copyright
© 2022, Coulombe
This article is distributed under the terms of the Creative Commons Attribution License, which permits unrestricted use and redistribution provided that the original author and source are credited.
Metrics
-
- 1,207
- views
-
- 120
- downloads
-
- 0
- citations
Views, downloads and citations are aggregated across all versions of this paper published by eLife.
Download links
Downloads (link to download the article as PDF)
Open citations (links to open the citations from this article in various online reference manager services)
Cite this article (links to download the citations from this article in formats compatible with various reference manager tools)
Further reading
-
- Cancer Biology
- Cell Biology
Understanding the cell cycle at the single-cell level is crucial for cellular biology and cancer research. While current methods using fluorescent markers have improved the study of adherent cells, non-adherent cells remain challenging. In this study, we addressed this gap by combining a specialized surface to enhance cell attachment, the FUCCI(CA)2 sensor, an automated image analysis pipeline, and a custom machine learning algorithm. This approach enabled precise measurement of cell cycle phase durations in non-adherent cells. This method was validated in acute myeloid leukemia cell lines NB4 and Kasumi-1, which have unique cell cycle characteristics, and we tested the impact of cell cycle-modulating drugs on NB4 cells. Our cell cycle analysis system, which is also compatible with adherent cells, is fully automated and freely available, providing detailed insights from hundreds of cells under various conditions. This report presents a valuable tool for advancing cancer research and drug development by enabling comprehensive, automated cell cycle analysis in both adherent and non-adherent cells.
-
- Cell Biology
- Developmental Biology
In most murine species, spermatozoa exhibit a falciform apical hook at the head end. The function of the sperm hook is not yet clearly understood. In this study, we investigate the role of the sperm hook in the migration of spermatozoa through the female reproductive tract in Mus musculus (C57BL/6), using a deep tissue imaging custom-built two-photon microscope. Through live reproductive tract imaging, we found evidence indicating that the sperm hook aids in the attachment of spermatozoa to the epithelium and facilitates interactions between spermatozoa and the epithelium during migration in the uterus and oviduct. We also observed synchronized sperm beating, which resulted from the spontaneous unidirectional rearrangement of spermatozoa in the uterus. Based on live imaging of spermatozoa-epithelium interaction dynamics, we propose that the sperm hook plays a crucial role in successful migration through the female reproductive tract by providing anchor-like mechanical support and facilitating interactions between spermatozoa and the female reproductive tract in the house mouse.