Flowering: Keeping it cool
The timing of flowering is one of the most important transitions during the life of a plant. Synchronising the flowering period with warmer temperatures enhances the reproductive success of a plant (and its impact on pollinators). To do so, plants must be able to sense cold temperatures, distinguish between short- and long-term periods of cold, and remember when these changes take place. This requires a complex interplay between internal regulators and environmental cues (Simpson and Dean, 2002; Wellmer and Riechmann, 2010).
In some plants, such as the model plant Arabidopsis thaliana, prolonged periods of cold lasting several weeks are required to initiate flowering via a process known as vernalisation. During this time, a gene called Flowering Locus C (FLC), which acts as a brake to flowering, gets switched off by epigenetic processes that stably hold the gene in the off position long after the vernalisation period (Michaels and Amasino, 1999; Sheldon et al., 1999; Michaels and Amasino, 2000; Whittaker and Dean, 2017).
Several decades of intensive research using a wide range of techniques has led to a well-established model for how the flowering block imposed by FLC is regulated. A long, non-coding RNA, called COOLAIR, is thought to play an important role in this model. Since COOLAIR is an antisense RNA with a complementary sequence of nucleotides to FLC, it is assumed to be an ideal candidate for suppressing the expression of this gene. Now, in eLife, Ilha Lee and colleagues – including Myeongjune Jeon and Goowon Jeong as joint first authors – report new results that challenge this theory (Jeon et al., 2023).
To better understand the role of COOLAIR in vernalisation, the researchers – who are based at the Seoul National University, the Chinese Academy of Sciences, and the Peking University Institute of Advanced Agricultural Sciences – did a combination of molecular, genetic and physiology experiments before, during and after vernalisation in A. thaliana plants and cells to explore the role of COOLAIR and some DNA transcription factors, known as CBFs, in vernalisation.
In a search for factors involved in the early stages of the vernalisation process, Jeon et al. focussed on the cold signal transduction that regulates the transcription of COOLAIR. The results revealed that the CBFs are required for COOLAIR transcription (Figure 1). CBF proteins have been known to play a central role for plants to increase their tolerance to freezing but they had not been linked to the vernalisation response before. Moreover, the CBF-binding DNA sequence in the COOLAIR promoter (a critical sequence for transcription) is conserved amongst different plant species, suggesting the CBF-COOLAIR regulator mechanism evolved some significant time ago.
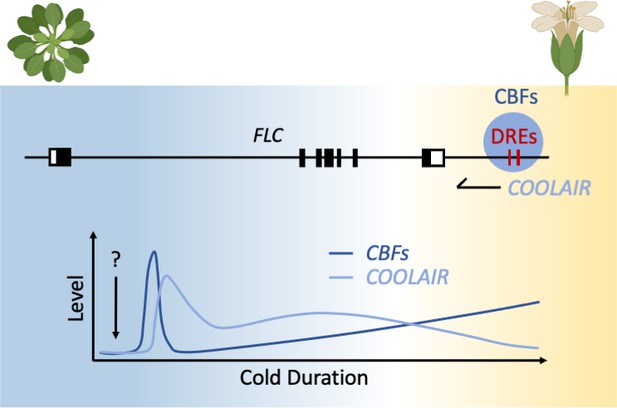
Vernalisation in Arabidopsis thaliana.
Some plants need a prolonged period of cold lasting several weeks to permit flowering in a process known as vernalisation. In A. thaliana, a gene called FLC stops plants from flowering during winter. During this time, FLC is highly expressed (black bars), but this activity is reduced after vernalisation. Jeong et al. show that early in the vernalisation process, CBF proteins (dark blue) bind to conserved DNA sequences (DREs) at the end of FLC to transcribe a long non-coding RNA, called COOLAIR (light blue). During vernalisation, the amount of CBFs increases, while COOLAIR levels decrease. The upstream regulator of CBF transcription remains unknown (shown as question mark).
In genetically modified plants that only had inactive forms of the genes coding for CBFs, cold-induced expression of COOLAIR was severely impaired. In modified plants with overactive CBF-coding genes, COOLAIR levels were high even when temperatures were warm. Unexpectedly, Jeon et al. found that these genetically modified plants were still able to go through vernalisation, even though they were unable to produce COOLAIR during the cold period. In addition, well described epigenetic histone modifications responsible for switching off the FLC gene remained unchanged before and after vernalisation in the genetically modified plants. This suggest that neither COOLAIR, nor the CBF transcription factors that transcribe COOLAIR, are required to induce vernalisation.
Jeon et al. used an elegant combination of genetic and molecular experiments to paint a compelling picture that suggests that the previously well-established vernalisation model requires updating. This is also in accordance with recent research, suggesting a similar theory (Helliwell et al., 2011; Luo et al., 2019). There are only a few genes where an in-depth analysis of how they are controlled has improved our overall knowledge of gene regulation. These include the trp operon genes in Escherichia coli, which regulate synthesis of the essential amino acid tryptophan and the beta-globin locus in mammals, famous for their involvement in the transport of oxygen (Yanofsky, 1981; Myers et al., 1986). It is clear the FLC locus also deserves a spot on this list.
References
-
Memories of winter: vernalization and the competence to flowerPlant, Cell and Environment 23:1145–1153.https://doi.org/10.1046/j.1365-3040.2000.00643.x
-
Gene networks controlling the initiation of flower developmentTrends in Genetics 26:519–527.https://doi.org/10.1016/j.tig.2010.09.001
-
The FLC locus: a platform for discoveries in epigenetics and adaptationAnnual Review of Cell and Developmental Biology 33:555–575.https://doi.org/10.1146/annurev-cellbio-100616-060546
Article and author information
Author details
Publication history
Copyright
© 2023, Nguyen and Searle
This article is distributed under the terms of the Creative Commons Attribution License, which permits unrestricted use and redistribution provided that the original author and source are credited.
Metrics
-
- 630
- views
-
- 76
- downloads
-
- 1
- citations
Views, downloads and citations are aggregated across all versions of this paper published by eLife.
Download links
Downloads (link to download the article as PDF)
Open citations (links to open the citations from this article in various online reference manager services)
Cite this article (links to download the citations from this article in formats compatible with various reference manager tools)
Further reading
-
- Chromosomes and Gene Expression
- Microbiology and Infectious Disease
Candida glabrata can thrive inside macrophages and tolerate high levels of azole antifungals. These innate abilities render infections by this human pathogen a clinical challenge. How C. glabrata reacts inside macrophages and what is the molecular basis of its drug tolerance are not well understood. Here, we mapped genome-wide RNA polymerase II (RNAPII) occupancy in C. glabrata to delineate its transcriptional responses during macrophage infection in high temporal resolution. RNAPII profiles revealed dynamic C. glabrata responses to macrophages with genes of specialized pathways activated chronologically at different times of infection. We identified an uncharacterized transcription factor (CgXbp1) important for the chronological macrophage response, survival in macrophages, and virulence. Genome-wide mapping of CgXbp1 direct targets further revealed its multi-faceted functions, regulating not only virulence-related genes but also genes associated with drug resistance. Finally, we showed that CgXbp1 indeed also affects fluconazole resistance. Overall, this work presents a powerful approach for examining host-pathogen interaction and uncovers a novel transcription factor important for C. glabrata’s survival in macrophages and drug tolerance.
-
- Chromosomes and Gene Expression
- Neuroscience
Pathogenic variants in subunits of RNA polymerase (Pol) III cause a spectrum of Polr3-related neurodegenerative diseases including 4H leukodystrophy. Disease onset occurs from infancy to early adulthood and is associated with a variable range and severity of neurological and non-neurological features. The molecular basis of Polr3-related disease pathogenesis is unknown. We developed a postnatal whole-body mouse model expressing pathogenic Polr3a mutations to examine the molecular mechanisms by which reduced Pol III transcription results primarily in central nervous system phenotypes. Polr3a mutant mice exhibit behavioral deficits, cerebral pathology and exocrine pancreatic atrophy. Transcriptome and immunohistochemistry analyses of cerebra during disease progression show a reduction in most Pol III transcripts, induction of innate immune and integrated stress responses and cell-type-specific gene expression changes reflecting neuron and oligodendrocyte loss and microglial activation. Earlier in the disease when integrated stress and innate immune responses are minimally induced, mature tRNA sequencing revealed a global reduction in tRNA levels and an altered tRNA profile but no changes in other Pol III transcripts. Thus, changes in the size and/or composition of the tRNA pool have a causal role in disease initiation. Our findings reveal different tissue- and brain region-specific sensitivities to a defect in Pol III transcription.