Symbiosis: How corals get their nutrients
Living inside corals are a family of algae known as Symbiodiniaceae, which provide nutrients that corals need to survive (LaJeunesse et al., 2018). However, rising water temperatures are putting corals under stress, causing them to eject their algae and lose their color. Dramatic images of this heat-driven coral reef ‘bleaching’ have drawn attention to the breakdown of the symbiotic relationship between coral animals and their algae. With entire ecosystems relying on this close-knit relationship, it is important to understand how corals and algae interact and how this might be altered by changing environmental conditions.
The algae reside within an acidic compartment inside coral cells where they can perform photosynthesis (Barott et al., 2015). They use sunlight to fix carbon from the environment and transfer the resulting energy-rich nutrients – such as lipids, amino acids and sugars – to their coral hosts (Rosset et al., 2021; Figure 1A). Glucose is one of the key sugars transferred (Burriesci et al., 2012), which coral cells then take up using specialized transporters, such as those in the GLUT family (Sproles et al., 2018). But how the sugars get out of algae in the first place is largely unknown. Previous studies have suggested that algae may release glucose using transporter proteins that respond to sugar concentrations in the environment (Xiang et al., 2018). Now, in eLife, Shinichiro Maruyama (Tohoku University and Ochanomizu University) and colleagues from multiple institutes in Japan – including Yuu Ishii as first author – report that algae can also secrete sugars by degrading their cell walls (Ishii et al., 2023).
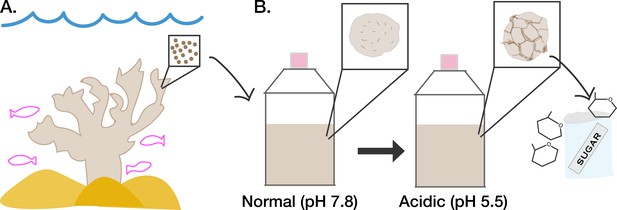
Acidic conditions affect how symbiotic algae secrete sugar.
(A) A family of algae known as Symbiodiniaceae (represented as brown circles in inset) live within reef-building corals (beige) where they transfer nutrients they have fixed via photosynthesis to their hosts. (B) The symbiotic algae can also grow in culture under normal conditions (pH 7.8, left), in which they display a smooth appearance (depicted in left inset). When the culture medium of the algae is made more acidic (pH 5.5) to mimic the internal environment of coral (right), the algae display a more wrinkled cell surface (depicted in right inset). The wrinkled cell surface is due to the algae digesting their own cell walls using activated cellulase enzymes. This results in increased secretion of sugars into the surrounding environment that can then be taken up by coral cells.
The team studied a species of algae from the Symbiodiniaceae family known as Breviolum minutum which were living freely outside of a host. To recreate the environment algae experience inside coral cells, the pH of the medium the algae were cultured in was changed from normal sea water levels (pH 7.8) to a more acidic formula (pH 5.5). This decreased the rate at which algae grew and photosynthesized, which led Ishii et al. to believe that nutrient transfer would also be inhibited. Instead, they found that the algae were actually secreting more glucose as well as another sugar called galactose. Chemically blocking photosynthesis with a targeted inhibitor also increased sugar output, contradicting previous expectations that this process is required for nutrient transfer (Burriesci et al., 2012).
To explain these findings, Ishii et al. used scanning electron microscopy to take detailed images of the surfaces of the algae, which have been shown to respond to glucose in the environment (Xiang et al., 2018). This revealed that under acidic conditions, algae surfaces changed from mostly smooth to mostly wrinkled (Figure 1B). Interestingly, the wrinkle pattern appeared to correspond to the sites where the plates that make up the algae cell wall join together (LaJeunesse et al., 2018), suggesting that acid affects these weaker junctions between plates first. Transmission electron scanning microscopy showed that the interior organization of the algae was also disrupted, with the cell wall pulling away from the body of the cell.
To understand how acidity increases sugar output and cell wall degradation, Ishii et al. compared the genes algae switched on when cultured in normal versus acidic conditions. Out of the genes that were only switched on in acidic conditions, several encoded proteins which help to break down carbohydrates. These included the genes for cellulases, enzymes that digest cellulose, which is a key component of the cell wall in algae and plants. Bringing these threads together, Ishii et al. blocked cellulase activity with a targeted drug, and found that this decreased the algae’s glucose and galactose outputs.
The results reveal a pathway by which symbiotic algae degrade their cell walls to release key nutrients, which can then be taken up by their coral host. Importantly, Ishii et al. point out that a combination of both digestion- and transporter-driven secretion are likely involved in sugar output. Transporter-driven secretion has been suggested to take around an hour and to rely on photosynthesis (Burriesci et al., 2012). Meanwhile, the digestion method discovered by Ishii et al. is slower (one day) and independent of sunlight, suggesting that it may mobilize stored materials when photosynthesis is not possible (e.g. in darkness). The symbiotic system may therefore be tunable according to the dynamic conditions of the coral reef.
While culture conditions of free-living algae may not always perfectly recreate the complex interior of a host cell (Maruyama and Weis, 2021), these results likely also apply to algae living in coral. Indeed, Ishii et al. found published datasets showing that algae cultured in normal (non-acidic) conditions regulate a key cellulase gene differently compared to symbiotic algae, supporting their finding that these cellulases are activated by acidic environments including those in coral cells (Xiang et al., 2020).
Future studies could examine the sugar export mechanisms of other species of symbiotic algae, particularly those which have been shown to influence the cellular acidity and bleaching tolerance of their coral hosts (Allen-Waller and Barott, 2023). As ocean temperatures continue to rise, these kinds of investigations are more important than ever.
References
-
Evidence that glucose is the major transferred metabolite in dinoflagellate-cnidarian symbiosisThe Journal of Experimental Biology 215:3467–3477.https://doi.org/10.1242/jeb.070946
-
The molecular language of the cnidarian-dinoflagellate symbiosisTrends in Microbiology 29:320–333.https://doi.org/10.1016/j.tim.2020.08.005
-
Phylogenetic characterization of transporter proteins in the cnidarian-dinoflagellate symbiosisMolecular Phylogenetics and Evolution 120:307–320.https://doi.org/10.1016/j.ympev.2017.12.007
Article and author information
Author details
Publication history
- Version of Record published: August 18, 2023 (version 1)
Copyright
© 2023, Hambleton
This article is distributed under the terms of the Creative Commons Attribution License, which permits unrestricted use and redistribution provided that the original author and source are credited.
Metrics
-
- 974
- views
-
- 67
- downloads
-
- 1
- citations
Views, downloads and citations are aggregated across all versions of this paper published by eLife.
Download links
Downloads (link to download the article as PDF)
Open citations (links to open the citations from this article in various online reference manager services)
Cite this article (links to download the citations from this article in formats compatible with various reference manager tools)
Further reading
-
- Evolutionary Biology
Extant ecdysozoans (moulting animals) are represented by a great variety of soft-bodied or articulated organisms that may or may not have appendages. However, controversies remain about the vermiform nature (i.e. elongated and tubular) of their ancestral body plan. We describe here Beretella spinosa gen. et sp. nov. a tiny (maximal length 3 mm) ecdysozoan from the lowermost Cambrian, Yanjiahe Formation, South China, characterized by an unusual sack-like appearance, single opening, and spiny ornament. Beretella spinosa gen. et sp. nov has no equivalent among animals, except Saccorhytus coronarius, also from the basal Cambrian. Phylogenetic analyses resolve both fossil species as a sister group (Saccorhytida) to all known Ecdysozoa, thus suggesting that ancestral ecdysozoans may have been non-vermiform animals. Saccorhytids are likely to represent an early off-shot along the stem-line Ecdysozoa. Although it became extinct during the Cambrian, this animal lineage provides precious insight into the early evolution of Ecdysozoa and the nature of the earliest representatives of the group.
-
- Biochemistry and Chemical Biology
- Evolutionary Biology
The emergence of new protein functions is crucial for the evolution of organisms. This process has been extensively researched for soluble enzymes, but it is largely unexplored for membrane transporters, even though the ability to acquire new nutrients from a changing environment requires evolvability of transport functions. Here, we demonstrate the importance of environmental pressure in obtaining a new activity or altering a promiscuous activity in members of the amino acid-polyamine-organocation (APC)-type yeast amino acid transporters family. We identify APC members that have broader substrate spectra than previously described. Using in vivo experimental evolution, we evolve two of these transporter genes, AGP1 and PUT4, toward new substrate specificities. Single mutations on these transporters are found to be sufficient for expanding the substrate range of the proteins, while retaining the capacity to transport all original substrates. Nonetheless, each adaptive mutation comes with a distinct effect on the fitness for each of the original substrates, illustrating a trade-off between the ancestral and evolved functions. Collectively, our findings reveal how substrate-adaptive mutations in membrane transporters contribute to fitness and provide insights into how organisms can use transporter evolution to explore new ecological niches.