Germ Cells: From stem cell to egg cell
Most stem cells are able to differentiate into a wide variety of cell types in a given organ system for the purposes of regeneration and repair (Morrison and Spradling, 2008). In the blood, for example, hematopoietic stem cells can give rise to red blood cells, various types of white blood cell, and a range of other blood cell types (Seita and Weissman, 2010). On the other hand, in the reproductive system, “germline” stem cells give rise to just two cell types – egg cells and sperm cells (Lehmann, 2012). However, when a sperm cell fertilizes an egg cell, the resulting zygote has the potential to give rise to an entire organism containing a large variety of cell types.
As a germline stem cell transitions into an egg cell, a delicate balance must be struck: genes specific to the stem cell must be silenced, and egg-specific genes must be activated. Once activated, these genes have a pivotal role in directing egg formation and ensuring a smooth transition from a germline stem cell to an egg cell. However, the mechanisms through which reproductive stem cells regulate this potential remain largely unknown.
Initially, it was believed that there existed a simple "on-off switch" between the stem cell and egg-specific programs. However, recent research has challenged this notion by showing that most of the genes essential for egg formation and development are expressed continuously during the stem cell stage, albeit at lower levels (Blatt et al., 2021; Sarkar et al., 2023; DeLuca et al., 2020). This suggests that, genetically, the egg program is always active in the mature reproductive organs, with stem cells restricting their potential to become eggs by ensuring that these genes are not expressed (Blatt et al., 2020; Slaidina and Lehmann, 2014). Thus, for genes that promote egg development, regulation is more similar to a "volume control" than a binary "on-off" switch.
Now, in eLife, Allan Spradling and colleagues at the Carnegie Institution for Science – including Liang-Yu Pang as first author – report the results of experiments on female fruit flies that reveal more details about how a germline stem cell becomes an egg cell (Figure 1; Pang et al., 2023).
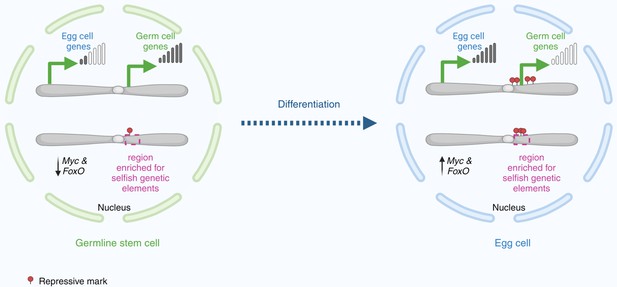
Changes in gene expression as a germline stem cell becomes an egg cell.
In the nucleus of a germline stem cell (left), germ cell genes near the centre of the chromosome – adjacent to a region enriched with selfish genetic elements – are expressed at high levels. Conversely, egg cell genes and the transcription factors Myc and FoxO are transcribed at lower levels. As the germline stem cell differentiates to become an egg cell (right), Myc and FoxO levels increase to meet the metabolic demands required by the egg cell as it grows. In the nucleus, the egg cell genes are expressed at high levels, whereas the germ cell genes are silenced by histone modifications (red circles).
First, Pang et al. compared the gene expression of germline stem cells and egg cells. RNA sequencing revealed that most of the genes expressed in egg cells were also expressed at the earlier stage. However, the diversity of expressed genes decreased as the germline stem cells became egg cells.
Genes can be silenced when histones – proteins that interact with DNA and package it into a structure called chromatin – are modified by, for example, the addition of methyl groups to an amino acid residue in the histone. These modifications or ‘marks’ change how accessible genes are to the molecular machinery required to express them, and therefore regulate gene expression. Two marks, known as H3K9me3 and H3K27me3, are associated with gene silencing. A unit of chromatin contains eight histones, and H3K9me3 means that a histone called H3 has been modified by the addition of three methyl groups to the 9th lysine (K) residue of this histone.
Using a combination of fluorescent imaging and a technique called ChIP-Seq, Pang et al. found that the number of both marks increase during the transition from germline stem cell to egg cell. Notably, the number of H3K9me3 marks increased in regions next to genes that support the stem cell program, and next to selfish genetic elements that can cause damage to the genome when activated. Loss of the enzyme responsible for depositing these marks upregulates genes supporting stem cell characteristics (Rangan et al., 2011; Sarkar et al., 2023; Sienski et al., 2015) and is required for proper egg production and fertility (DeLuca et al., 2020; Rangan et al., 2011). Similarly, loss of the enzymes required for H3K27me3 marks also upregulates stem cell genes. Taken together, these findings show that both H3K9me3 and H3K27me3 chromatin marks are necessary to completely silence the stem cell program and allow the transition to the egg cell to take place.
Egg growth requires significant metabolic changes and increased gene expression (Sieber and Spradling, 2017). Pang et al. observed upregulation of two transcription factors linked to increased metabolism and gene expression, Myc and FoxO, after the egg cell had formed. There were also large changes to mitochondrial metabolism that facilitated enhanced production of amino acids, nucleotides, carbohydrates, and lipids to support the substantial growth of the egg. Therefore, the transition from germline stem cell to egg cell is also accompanied by metabolic rewiring.
The work of Pang et al. sheds new light on our understanding of this transition from germline stem cell to egg cell, and raises further questions: we still do not know the identity of the genes responsible for orchestrating the metabolic shift, or fully understand the mechanisms that drive the upregulation of the egg program. Furthermore, it remains a mystery how stem cells exert control over selfish genetic elements when they are in an open chromatin state and able to be expressed. Research in this direction promises to unveil deeper insights into how stem cells modulate their ability to differentiate.
References
-
Post-transcriptional gene regulation regulates germline stem cell to oocyte transition during Drosophila oogenesisCurrent Topics in Developmental Biology 140:3–34.https://doi.org/10.1016/bs.ctdb.2019.10.003
-
Germline stem cells: origin and destinyCell Stem Cell 10:729–739.https://doi.org/10.1016/j.stem.2012.05.016
-
piRNA production requires heterochromatin formation in DrosophilaCurrent Biology 21:1373–1379.https://doi.org/10.1016/j.cub.2011.06.057
-
Hematopoietic stem cell: self-renewal versus differentiationWiley Interdisciplinary Reviews. Systems Biology and Medicine 2:640–653.https://doi.org/10.1002/wsbm.86
-
The role of metabolic states in development and diseaseCurrent Opinion in Genetics & Development 45:58–68.https://doi.org/10.1016/j.gde.2017.03.002
-
Silencio/CG9754 connects the Piwi-piRNA complex to the cellular heterochromatin machineryGenes & Development 29:2258–2271.https://doi.org/10.1101/gad.271908.115
-
Translational control in germline stem cell developmentThe Journal of Cell Biology 207:13–21.https://doi.org/10.1083/jcb.201407102
Article and author information
Author details
Publication history
Copyright
© 2023, Kotb and Rangan
This article is distributed under the terms of the Creative Commons Attribution License, which permits unrestricted use and redistribution provided that the original author and source are credited.
Metrics
-
- 775
- views
-
- 86
- downloads
-
- 0
- citations
Views, downloads and citations are aggregated across all versions of this paper published by eLife.
Download links
Downloads (link to download the article as PDF)
Open citations (links to open the citations from this article in various online reference manager services)
Cite this article (links to download the citations from this article in formats compatible with various reference manager tools)
Further reading
-
- Stem Cells and Regenerative Medicine
The lateral wall of the mouse subventricular zone harbors neural stem cells (NSC, B cells) which generate proliferating transient-amplifying progenitors (TAP, C cells) that ultimately give rise to neuroblasts (NB, A cells). Molecular profiling at the single-cell level struggles to distinguish these different cell types. Here, we combined transcriptome analyses of FACS-sorted cells and single-cell RNAseq to demonstrate the existence of an abundant, clonogenic and multipotent population of immature neuroblasts (iNB cells) at the transition between TAP and migrating NB (mNB). iNB are reversibly engaged in neuronal differentiation. Indeed, they keep molecular features of both undifferentiated progenitors, plasticity and unexpected regenerative properties. Strikingly, they undergo important progressive molecular switches, including changes in the expression of splicing regulators leading to their differentiation in mNB subdividing them into two subtypes, iNB1 and iNB2. Due to their plastic properties, iNB could represent a new target for regenerative therapy of brain damage.
-
- Biochemistry and Chemical Biology
- Stem Cells and Regenerative Medicine
Human induced pluripotent stem cells (hiPSCs) have great potential to be used as alternatives to embryonic stem cells (hESCs) in regenerative medicine and disease modelling. In this study, we characterise the proteomes of multiple hiPSC and hESC lines derived from independent donors and find that while they express a near-identical set of proteins, they show consistent quantitative differences in the abundance of a subset of proteins. hiPSCs have increased total protein content, while maintaining a comparable cell cycle profile to hESCs, with increased abundance of cytoplasmic and mitochondrial proteins required to sustain high growth rates, including nutrient transporters and metabolic proteins. Prominent changes detected in proteins involved in mitochondrial metabolism correlated with enhanced mitochondrial potential, shown using high-resolution respirometry. hiPSCs also produced higher levels of secreted proteins, including growth factors and proteins involved in the inhibition of the immune system. The data indicate that reprogramming of fibroblasts to hiPSCs produces important differences in cytoplasmic and mitochondrial proteins compared to hESCs, with consequences affecting growth and metabolism. This study improves our understanding of the molecular differences between hiPSCs and hESCs, with implications for potential risks and benefits for their use in future disease modelling and therapeutic applications.