Anesthesia: Putting early sensory neurons to sleep
To better understand how animals sense their environment, studies measuring brain activity need to include neural recordings in awake behaving animals (Steinmetz et al., 2018; Chorev et al., 2009). However, such experiments are often difficult to conduct, and researchers also need to consider how the stimulus is applied and account for behaviors that may impact the stimulus being measured. Active sensation, such as sniffing or eye movements, can make it challenging to control and quantify a stimulus to the extent required for many analyses (Deschênes et al., 2012; Land, 1999).
Experiments in awake animals capture more natural behavior, while those conducted in anesthetized animals or acute brain slices allow sensory stimuli to be controlled more tightly. The latter can also identify more mechanistic details of the brain structures involved in sensory processing. Thus far, it has been widely assumed that neurons behave similarly enough across these different conditions so that insights from one type of experiment can be translated to another.
However, the effects of anesthesia (and brain slicing) on various parts of the central nervous system have been a topic of considerable attention (Hao et al., 2020). For example, cognitive behaviors, like attention and motivational state, which have a large impact on neural activity, are absent in anesthetized animals (Hembrook-Short et al., 2017; Rossi et al., 2013). But neurons in early sensory systems, such as the retina, already process much of the neural code before it is even transmitted to the brain visual centers. These early sensory systems thus receive limited feedback from the brain and are thought to be less affected by anesthesia (Gastinger et al., 2006). Now, in eLife, Tom Boissonnet, Matteo Tripodi and Hiroki Asari at the EMBL Rome and the Université Grenoble Alpes report new findings that challenge this assumption (Boissonnet et al., 2023).
Boissonnet et al. recorded and compared how the output neurons of the retina (the retinal ganglion cells) responded to light in awake animals, in anesthetized animals and in experimentally isolated retinas. Activity levels of the ganglion cells in the isolated retinas were recorded through spike recordings. For recordings in living animals, Boissonnet et al. inserted electrical probes directly into the optic tract, the nerve bundle that relays visual information to the brain. Boissonnet et al. then applied full-field light modulation to stimulate the entire retina with light of equal intensity to avoid the effects of eye movements, and also accounted for pupil constriction. This allowed them to give the retina nearly identical stimuli across the three conditions.
The study revealed that ganglion cells in awake animals were able to respond to substantially higher temporal frequencies of light stimuli than ganglion cells in an animal under anesthesia or ganglion cells in isolated retinas. Isolated retinas also showed markedly lower spiking activity (Figure 1).
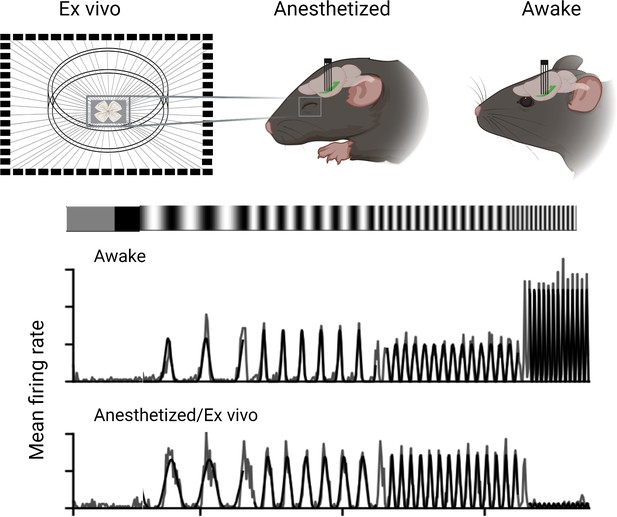
Experimental conditions affect the output of retinal ganglion cells in mice.
Boisonnet et al. studied the characteristics of retinal ganglion cells in mice by comparing how they respond in awake and anesthetized animals, as well as isolated retinal cells (ex vivo). The recordings in the live animals were carried out by placing an electrode into the optic tract and measuring their neural activity when exposed to a flickering light. These results showed that awake animals had faster and stronger responses to the light stimuli compared to anesthetized and isolated preparations.
Image credit: Created using Biorender and adapted from Figure 4 of the manuscript by Boissonnet et al., 2023.
As with many innovative approaches, the study of Boissonnet et al. raises at least as many new questions as it answers. Contrary to previous hypotheses, anesthesia appears to have profound effects even at the early stages of visual processing within the retina. From a mechanistic perspective, this is, perhaps, not terribly surprising. Both anesthetics used in the study are known to interact with a set of inhibitory receptors for the neurotransmitter GABA (γ-aminobutyric acid), and they are prevalent throughout the retina (Michelson and Kozai, 2018; Bharioke et al., 2022; Yang, 2004).
Still, the magnitude of the effect – the anesthetized preparation was substantially slower than even the isolated retina – is an important point to consider when interpreting recordings in the visual system of awake and anesthetized animals. Interestingly, a higher firing activity and faster response dynamics in awake animals have also been found in the parts of the brain that the axons of the retinal ganglion primarily project onto (that is, the dorsal lateral geniculate nucleus and the superior colliculus; Durand et al., 2016; De Franceschi and Solomon, 2018). The results of Boissonnet et al. suggest that at least some of these differences originate in the retina. Comparing data from live animals and isolated cells also comes with an experimental caveat. While Boissonnet et al. did their best to replicate light conditions and temperature between the different set-ups, it is likely that some sampling biases among the over 40 types of ganglion cells in mice were different in the awake compared to the isolated retinas (Goetz et al., 2022). This makes it difficult to interpret the pooled results across the different set-ups.
While this work is a critical first step, there is still a long way to go before it is possible to measure the response of retinal ganglion cells in a natural context. Boisonnet et al. did not measure spatial or movement-related responses. Instead they ensured that the stimuli were spatially uniform, and the animals were head-fixed to minimize their eye movement. Future research could build on this method to further refine the technique and ensure the behavioral contexts of the animal match how retinal ganglion cells work in natural conditions. It will also be interesting to see if other neurons in early sensory systems, particularly those in the peripheral nervous system, like the nose and ear, have comparable results to the retina, which is part of the central nervous system (Schumacher et al., 2011). For now, though, researchers should strongly consider the implications of putting early sensory neurons to sleep.
References
-
Electrophysiological recordings from behaving animals--going beyond spikesCurrent Opinion in Neurobiology 19:513–519.https://doi.org/10.1016/j.conb.2009.08.005
-
Visual response properties of neurons in the superficial layers of the superior colliculus of awake mouseThe Journal of Physiology 596:6307–6332.https://doi.org/10.1113/JP276964
-
Sniffing and whisking in rodentsCurrent Opinion in Neurobiology 22:243–250.https://doi.org/10.1016/j.conb.2011.11.013
-
Retinopetal axons in mammals: emphasis on histamine and serotoninCurrent Eye Research 31:655–667.https://doi.org/10.1080/02713680600776119
-
The effects of general anesthetics on synaptic transmissionCurrent Neuropharmacology 18:936–965.https://doi.org/10.2174/1570159X18666200227125854
-
Motion and vision: why animals move their eyesJournal of Comparative Physiology. A, Sensory, Neural, and Behavioral Physiology 185:341–352.https://doi.org/10.1007/s003590050393
-
Isoflurane and ketamine differentially influence spontaneous and evoked laminar electrophysiology in mouse V1Journal of Neurophysiology 120:2232–2245.https://doi.org/10.1152/jn.00299.2018
-
Challenges and opportunities for large-scale electrophysiology with Neuropixels probesCurrent Opinion in Neurobiology 50:92–100.https://doi.org/10.1016/j.conb.2018.01.009
-
Characterization of receptors for glutamate and GABA in retinal neuronsProgress in Neurobiology 73:127–150.https://doi.org/10.1016/j.pneurobio.2004.04.002
Article and author information
Author details
Publication history
Copyright
© 2023, Fadjukov and Schwartz
This article is distributed under the terms of the Creative Commons Attribution License, which permits unrestricted use and redistribution provided that the original author and source are credited.
Metrics
-
- 562
- views
-
- 56
- downloads
-
- 0
- citations
Views, downloads and citations are aggregated across all versions of this paper published by eLife.
Download links
Downloads (link to download the article as PDF)
Open citations (links to open the citations from this article in various online reference manager services)
Cite this article (links to download the citations from this article in formats compatible with various reference manager tools)
Further reading
-
- Neuroscience
Chronic pain is a prevalent and debilitating condition whose neural mechanisms are incompletely understood. An imbalance of cerebral excitation and inhibition (E/I), particularly in the medial prefrontal cortex (mPFC), is believed to represent a crucial mechanism in the development and maintenance of chronic pain. Thus, identifying a non-invasive, scalable marker of E/I could provide valuable insights into the neural mechanisms of chronic pain and aid in developing clinically useful biomarkers. Recently, the aperiodic component of the electroencephalography (EEG) power spectrum has been proposed to represent a non-invasive proxy for E/I. We, therefore, assessed the aperiodic component in the mPFC of resting-state EEG recordings in 149 people with chronic pain and 115 healthy participants. We found robust evidence against differences in the aperiodic component in the mPFC between people with chronic pain and healthy participants, and no correlation between the aperiodic component and pain intensity. These findings were consistent across different subtypes of chronic pain and were similarly found in a whole-brain analysis. Their robustness was supported by preregistration and multiverse analyses across many different methodological choices. Together, our results suggest that the EEG aperiodic component does not differentiate between people with chronic pain and healthy individuals. These findings and the rigorous methodological approach can guide future studies investigating non-invasive, scalable markers of cerebral dysfunction in people with chronic pain and beyond.
-
- Neuroscience
Biological memory networks are thought to store information by experience-dependent changes in the synaptic connectivity between assemblies of neurons. Recent models suggest that these assemblies contain both excitatory and inhibitory neurons (E/I assemblies), resulting in co-tuning and precise balance of excitation and inhibition. To understand computational consequences of E/I assemblies under biologically realistic constraints we built a spiking network model based on experimental data from telencephalic area Dp of adult zebrafish, a precisely balanced recurrent network homologous to piriform cortex. We found that E/I assemblies stabilized firing rate distributions compared to networks with excitatory assemblies and global inhibition. Unlike classical memory models, networks with E/I assemblies did not show discrete attractor dynamics. Rather, responses to learned inputs were locally constrained onto manifolds that ‘focused’ activity into neuronal subspaces. The covariance structure of these manifolds supported pattern classification when information was retrieved from selected neuronal subsets. Networks with E/I assemblies therefore transformed the geometry of neuronal coding space, resulting in continuous representations that reflected both relatedness of inputs and an individual’s experience. Such continuous representations enable fast pattern classification, can support continual learning, and may provide a basis for higher-order learning and cognitive computations.