Sugar is an endogenous cue for juvenile-to-adult phase transition in plants
Abstract
The transition from the juvenile to adult phase in plants is controlled by diverse exogenous and endogenous cues such as age, day length, light, nutrients, and temperature. Previous studies have shown that the gradual decline in microRNA156 (miR156) with age promotes the expression of adult traits. However, how age temporally regulates the abundance of miR156 is poorly understood. We show here that the expression of miR156 responds to sugar. Sugar represses miR156 expression at both the transcriptional level and post-transcriptional level through the degradation of miR156 primary transcripts. Defoliation and photosynthetic mutant assays further demonstrate that sugar from the pre-existing leaves acts as a mobile signal to repress miR156, and subsequently triggers the juvenile-to-adult phase transition in young leaf primordia. We propose that the gradual increase in sugar after seed germination serves as an endogenous cue for developmental timing in plants.
https://doi.org/10.7554/eLife.00269.001eLife digest
Like animals, plants go through several stages of development before they reach maturity, and it has long been thought that some of the transitions between these stages are triggered by changes in the nutritional status of the plant. Now, based on experiments with the plant Arabidopsis thaliana, Yu et al. and, independently, Yang et al. have provided fresh insights into the role of sugar in ‘vegetative phase change'—the transition from the juvenile form of a plant to the adult plant.
The new work takes advantage of the fact that vegetative phase change is controlled by two genes that encode microRNAs (MIRNAs). Arabidopsis has eight MIR156 genes and both groups confirmed that supplying plants with sugar reduces the expression of two of these—MIR156A and MIR156C—while sugar deprivation increases their expression. Removing leaves also leads to upregulation of both genes, and delays the juvenile-to-adult transition. Given that this effect can be partially reversed by providing the plant with sugar, it is likely that sugar produced in the leaves—or one of its metabolites—is the signal that triggers the juvenile-to-adult transition through the reduction of miR156 levels.
Yu and co-workers confirmed that sugar also reduces the expression of MIR156 in tobacco, moss, and tomato plants, suggesting that this mechanism is evolutionarily conserved. Consistent with the work of Yang and colleagues, Yu and co-workers revealed that sugar is able to reduce the transcription of MIR156A and MIR156C genes into messenger RNA. Moreover, they showed that sugar can also suppress MIR156 expression by promoting the breakdown of MIR156A and MIR156C primary messenger RNA transcripts.
The work of Yu et al. and Yang et al. has thus provided key insights into the mechanisms by which a leaf-derived signal controls a key developmental change in plants. Just as fruit flies use their nutritional status to regulate the onset of metamorphosis, and mammals use it to control the onset of puberty, so plants use the level of sugar in their leaves to trigger the transition from juvenile to adult forms.
https://doi.org/10.7554/eLife.00269.002Introduction
After seed germination, plants undergo two developmental transitions: juvenile-to-adult and adult-to-reproductive (Bäurle and Dean, 2006). The transition from the juvenile to adult phase is marked by acquisition of reproductive competence and changes in leaf morphology (Poethig, 2010). The adult to reproductive transition, also known as flowering, transforms the identity of the shoot apical meristem from vegetative into inflorescence. Physiological and genetic studies have demonstrated that both developmental transitions are regulated not only by environmental signals such as day length, light intensity, and ambient temperature, but also by endogenous signals transmitted by plant hormones and age.
microRNA156 (miR156), which targets SQUAMOSA PROMOTER BINDING PROTEIN-LIKE (SPL) transcriptional factors, provides an endogenous age cue for developmental timing in plants (Poethig, 2010). The expression of miR156 decreases over time, with a concomitant rise in SPL level (Wu and Poethig, 2006; Wang et al., 2009). Overexpression of miR156 prolongs the juvenile phase, whereas a reduction in miR156 level results in an accelerated expression of adult traits (Wu and Poethig, 2006; Wu et al., 2009). SPL promotes the juvenile-to-adult phase transition and flowering through activation of miR172 and MADS-box genes (Wang et al., 2009; Wu et al., 2009; Yamaguchi et al., 2009; Jung et al., 2011). Very recently, defoliation experiments and expression analyses demonstrated that the repression of miR156 in the leaf primordia is mediated by a mobile signal(s) derived from the pre-existing leaves (Yang et al. 2011). However, the identity of this signal is still unknown.
In addition to being essential as prime carbon and energy sources, sugars also play critical roles as signaling molecules (Rolland and Sheen, 2005; Smeekens et al., 2010). In Arabidopsis thaliana, diverse sugar signals are perceived and transduced through a glucose sensor, HEXOKINASE1 (HXK1). HXK1 exerts its regulatory function through distinct molecular mechanisms including transcriptional activation, translational inhibition, mRNA decay, and protein degradation (Rolland and Sheen, 2005). Analyses of two catalytic inactive HXK1 alleles further indicate that the signaling activity of HXK1 is uncoupled from its catalytic activity (Moore et al., 2003). Recently, a nuclear HXK1 complex has been identified (Cho et al., 2006). In this complex, HXK1 binds to two unconventional partners, the vacuolar H+-ATPase B1 (VHA-B1) and the 19S regulatory particle of a proteasome subunit (RPT5B). Since neither VHA-B1 nor RPT5B has DNA binding capacity, the precise molecular mechanism by which this nuclear-localized HXK1 complex regulates gene expression remains unanswered. In addition to the HXK1-dependent pathway, some glucose-responsive genes are regulated through an HXK1-independent pathway. For instance, the expression of the genes encoding chalcone synthase, phenylalanine ammonia-lyase, and asparagine synthase responds to glucose signaling in the absence of HXK1 (Xiao et al., 2000).
Here, we performed expression and mutant analyses to identify the upstream regulator of miR156. Our results demonstrate that the expression of miR156 quickly responds to sugar. Sugar reduces miR156 abundance through both transcriptional repression and transcript degradation. Thus, gradual accumulation of sugar after seed germination leads to a reduced level of miR156, which promotes the juvenile-to-adult phase transition in plants.
Results
MIR156A and MIR156C have dominant roles within the MIR156 gene family
The transition from juvenile to adult phase in Arabidopsis is accompanied by changes in vegetative morphology. Under long day conditions, the wild type Arabidopsis plants switch from the juvenile to the adult phase from the fifth or sixth leaf. The juvenile leaves are round, smooth on their margins, and barely develop trichomes (leaf hairs) on the abaxial side (lower side). By contrast, the adult leaves are elongated, serrated, and produce abaxial trichomes (Wu et al., 2009).
In the Arabidopsis genome, miR156 is encoded by eight coding loci (MIR156A–MIR156H) (Reinhart et al., 2002). To understand which locus or loci play important roles within this gene family, we identified all available MIR156 transfer-DNA (T-DNA) knockout plants (Samson et al., 2002; Alonso et al., 2003; Woody et al., 2007; Figure 1A and supplementary file 1A). Due to functional redundancy, none of these mutants exhibited visible developmental defects (data not shown). One of the double mutants, mir156a mir156c, displayed a similar, but weak phenotype as the transgenic plant expressing a target mimicry from the constitutively active 35S promoter (35S::MIM156), which reduced miR156 activity (Figure 1D; Franco-Zorrilla et al., 2007; Todesco et al., 2010). RNA gel blot demonstrated that the amount of miR156 was moderately decreased in mir156a mir156c in comparison with the wild type (Figure 1B). Accordingly, the transcript levels of two miR156-target genes, SPL3 and SPL9, were much higher in mir156a mir156c than in the wild type (Figure 1C).
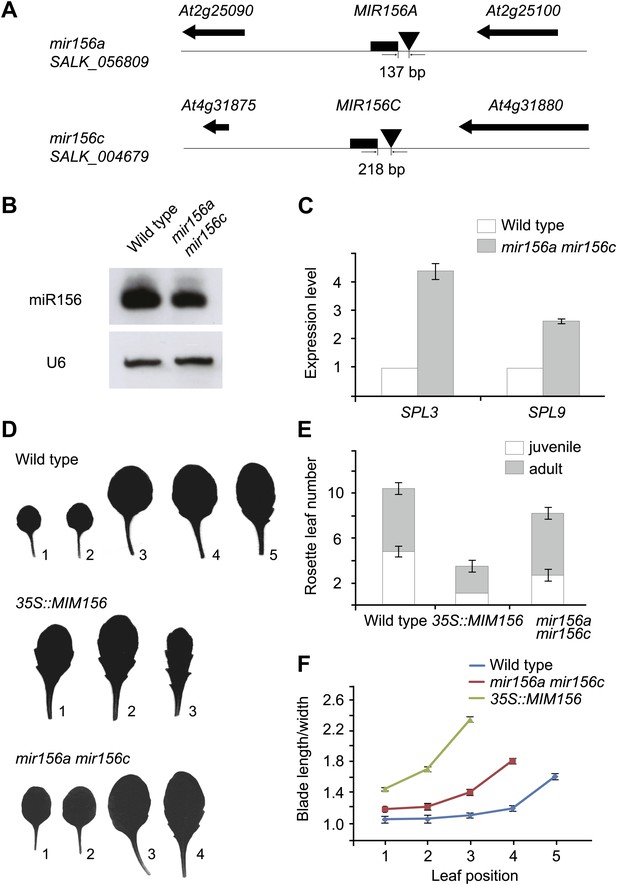
Phenotypic analyses of the mir156a mir156c double mutant.
(A) MIR156A and MIR156C genomic regions. Arrowheads mark T-DNA insertion sites. T-DNAs are inserted 137 bp and 218 bp upstream of the stem-loops of MIR156A and MIR156C, respectively. (B) Expression of miR156 in the wild type and the mir156a mir156c double mutant. U6 was monitored as loading control. (C) Expression of SPL3 and SPL9 in the wild type and the mir156a mir156c double mutant. The expression level in the wild type was set to 1.0. (D) Leaf morphology of wild type, mir156a mir156c, and 35S::MIM156 plants. The leaves were detached and scanned. The numbers indicate leaf positions. (E) The number of juvenile and adult leaves. n=12. (F) The length-to-width ratio of the blade. Fully expanded leaves were detached and scanned. The length and width of blades were measured. n=12. Error bars indicate SE.
Compared to the wild type, the mir156a mir156c mutant had a shortened juvenile phase. The appearance of abaxial trichomes in mir156a mir156c was accelerated by 2.1 plastochrons (Figure 1E). In addition, the length-to-width ratios of the blades in mir156a mir156c were much closer to those of the adult leaves in the wild type (Figure 1F). Furthermore, mir156a mir156c flowered earlier than the wild type (Figure 1E). Taken together, these results indicate that MIR156A and MIR156C have dominant roles within the miR156 family in Arabidopsis.
Sugar represses MIR156 expression
To elucidate the molecular mechanism by which the level of miR156 is regulated by age, we performed time course expression assays on miR156 and the primary transcripts of MIR156A and MIR156C (pri-MIR156A and pri-MIR156C) by RNA gel blot and quantitative real-time PCR (qRT-PCR). We collected plants grown under long day conditions for 8, 9, and 16 days. As previously reported, the abundance of miR156 gradually declined (Figure 2A,B; Wu and Poethig, 2006; Wang et al., 2009). Interestingly, the transcript levels of pri-MIR156A and pri-MIR156C, but not mature miR156, exhibited damped oscillations with the highest level in the morning and lowest before dark (Figure 2C,E; Figure 2—figure supplement 1). To test whether this expression pattern is generated by the circadian clock, we grew wild type plants for 5 days in long day conditions, and then transferred them to a constant light condition. After the transfer, the oscillating expression pattern of pri-MIR156A and pri-MIR156C was no longer observed (Figure 2D,F), demonstrating a negligible effect of the circadian clock on miR156 expression.
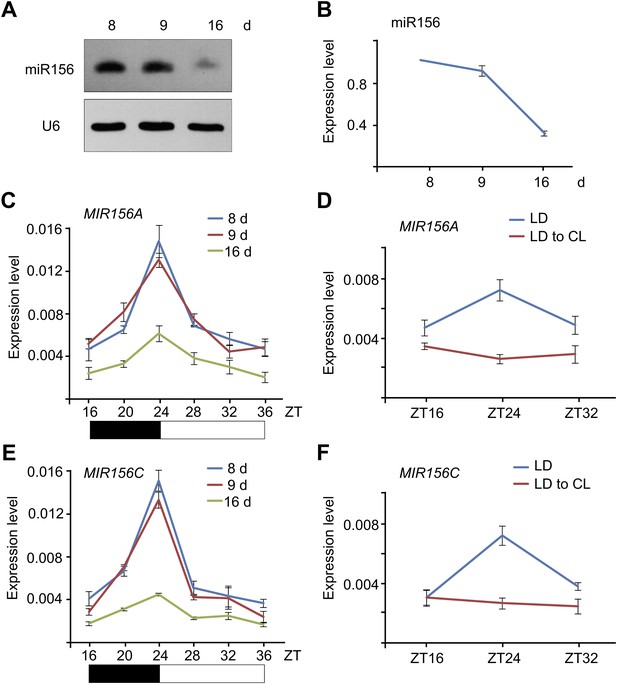
Expression of miR156.
(A and B) Accumulation of miR156 in 8-, 9-, and 16-day-old long day plants. Expression of miR156 was analyzed by small RNA blot (A) and qRT-PCR (B). The plants were collected at Zeitgeber time (ZT) 24. The expression level of miR156 in 8-day-old seedlings was set to 1. (C and E) Expression of pri-MIR156A (C) and pri-MIR156C (E). The plants were collected every 4 hr and subjected to qRT-PCR analyses. Black and white boxes indicate dark and light conditions, respectively. (D and F) Expression of pri-MIR156A (D) and pri-MIR156C (F) during the shift from long day (LD) to constant light (CL) conditions. Five-day-old wild type seedlings were shifted from long day to constant light conditions. The seedlings were collected at ZT 16, 24, and 32.
In addition to the circadian clock, endogenous carbohydrates are also able to trigger the oscillation of RNA transcripts (Bläsing et al., 2005). To test this possibility, we carried out sugar treatment assays. Five-day-old seedlings grown in 1/2 Murashige and Skoog (MS) liquid media were treated with sugars, including two disaccharides (maltose and sucrose) and two hexoses (glucose and fructose). The break-down of maltose results in two glucose molecules, whereas hydrolysis of sucrose produces glucose and fructose. The abundance of pri-MIR156A and pri-MIR156C was greatly reduced after 1 day of treatment with 50 mM sucrose, glucose, or maltose (Figure 3A). A reduction in pri-MIR156A or pri-MIR156C was not detected when the seedlings were treated with the same concentration of mannitol or 3-O-methyl-glucose (3-OMG), suggesting that the repression of pri-MIR156 by sugars is not due to osmotic stress. Consistent with the reduction in pri-MIR156 levels, mature miR156 was decreased after 1 day of sugar treatment (Figure 3A; Figure 3—figure supplement 1). Accordingly, the transcript levels of miR156-targeted genes, SPL9 and SPL15, were markedly increased (Figure 3B).
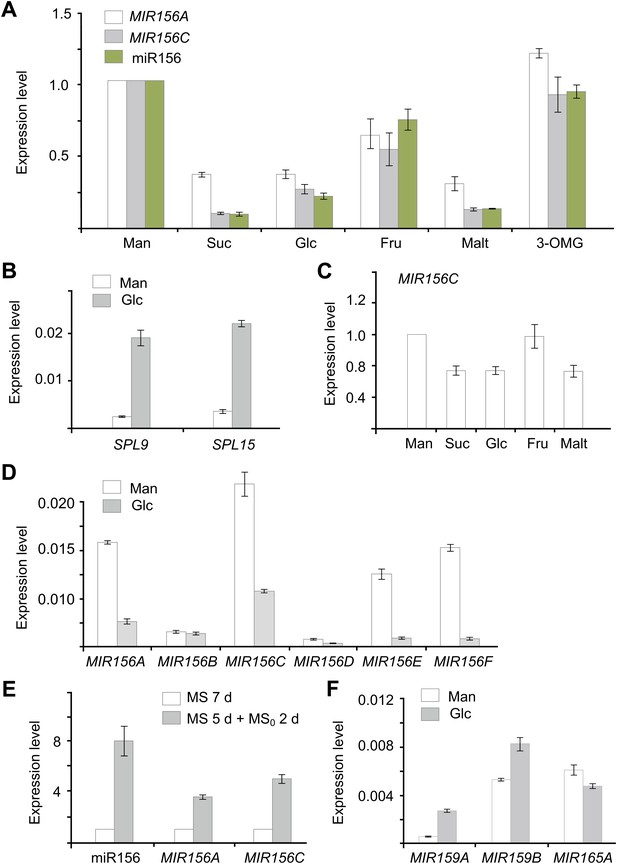
Sugar represses miR156.
(A) Expression of miR156, pri-MIR156A, and pri-MIR156C in response to sugar. Five-day-old wild type seedlings in 1/2 Murashige and Skoog (MS) liquid media were treated with 50 mM sucrose (Suc), glucose (Glc), fructose (Fru), maltose (Malt), or mannitol (Man) for 1 day. (B) Expression of SPL9 and SPL15 in response to sugar treatment. Five-day-old wild type seedlings were treated with 50 mM Man or Glc for 1 day. (C) pri-MIR156C quickly responds to sugar. Five-day-old wild type seedlings were treated with sugar for 30 min. The expression level in the mannitol-treated samples was set to 1. (D) Expression of pri-MIR156 transcripts. Five-day-old wild type seedlings in 1/2 MS liquid media were treated with 50 mM glucose or mannitol for 1 day. (E) Expression of miR156 and pri-MIR156C during sugar starvation. Five-day-old wild type seedlings in 1/2 MS liquid media supplemented with 50 mM sucrose were transferred to 1/2 MS media without sucrose (MS0). The seedlings were grown for another 2 days and then subjected to expression analyses. Seven-day-old seedlings in 1/2 MS liquid media supplemented with 50 mM sucrose were used as control. (F) Expression of other pri-MIRNA transcripts. Five-day-old wild type seedlings in 1/2 MS liquid media were treated with 50 mM glucose or mannitol for 1 day. The expression levels of pri-MIR156 and miR156 were normalized to those of TUBULIN (TUB). In the sugar treatment assays, 50 mM sugars were added at Zeitgeber time 12.
To monitor how fast miR156 responds to sugar, wild type seedlings were treated with glucose, sucrose, maltose, fructose, or mannitol for 30 min. A reduction of about 40% in pri-MIR156C was observed in the seedlings treated with glucose, sucrose, or maltose, while the level of pri-MIR156C was not altered in those treated with fructose or mannitol (Figure 3C). These results, together with the fact that glucose is the common hydrolytic product shared by sucrose and maltose, suggest that glucose plays a major role in repressing miR156.
To determine whether all the miR156 coding genes are repressed by sugar, we analyzed the expression of their primary transcripts. pri-MIR156G and pri-MIR156H were not readily amplified, probably due to their very low expression level (data not shown). The expression of other pri-MIR156 transcripts except pri-MIR156B was reduced after glucose treatment (Figure 3D).
To confirm the role of sugar in miR156 expression, we performed a sugar starvation experiment. Five-day-old wild type seedlings were transferred to 1/2 MS liquid media free of sugar and kept in the dark for 2 days. Compared to the seedlings grown in 1/2 MS liquid media supplemented with sugar under normal light conditions, the sugar-depleted seedlings exhibited a higher expression level of miR156 (Figure 3E).
To investigate whether sugar specifically represses miR156, we analyzed the expression of other miRNA primary transcripts, including pri-MIR159A, pri-MIR159B, and pri-MIR165A. The levels of all these transcripts were not reduced after sugar treatment (Figure 3F).
Sugar promotes the juvenile-to-adult phase transition
A recent study has shown that the juvenile-to-adult phase transition is mediated by a leaf-derived mobile signal that represses the expression of miR156 in young leaf primordia (Yang et al. 2011). Given the fact that sucrose is able to move within plants through the vascular tissues (Truernit 2001) and that sucrose as well as its hydrolytic product, glucose, repress the expression of miR156, we speculated that sugar is a potential candidate for this mobile signal. To test this hypothesis, we first investigated the relationship between sugar content and the level of miR156 in vivo. Under long day conditions, Arabidopsis plants show a rapid life cycle with very short juvenile and adult phases. For this reason, we grew wild type plants under short day conditions to extend the vegetative phase. Then 15-day-old (in the juvenile phase) and 60-day-old (in the adult phase) plants were collected at Zeitgeber time (ZT) 16. Expression analyses demonstrated that miR156 was highly abundant in 15-day-old plants but less so in 60-day-old plants (Figure 4A). In contrast to this expression pattern, 60-day-old plants exhibited a higher level of glucose, fructose, and sucrose than 15-day-old plants (Figure 4B). These results are consistent with our findings that sugar represses miR156 and indicate an inverse correlation between the level of miR156 and endogenous sugar content in vivo.
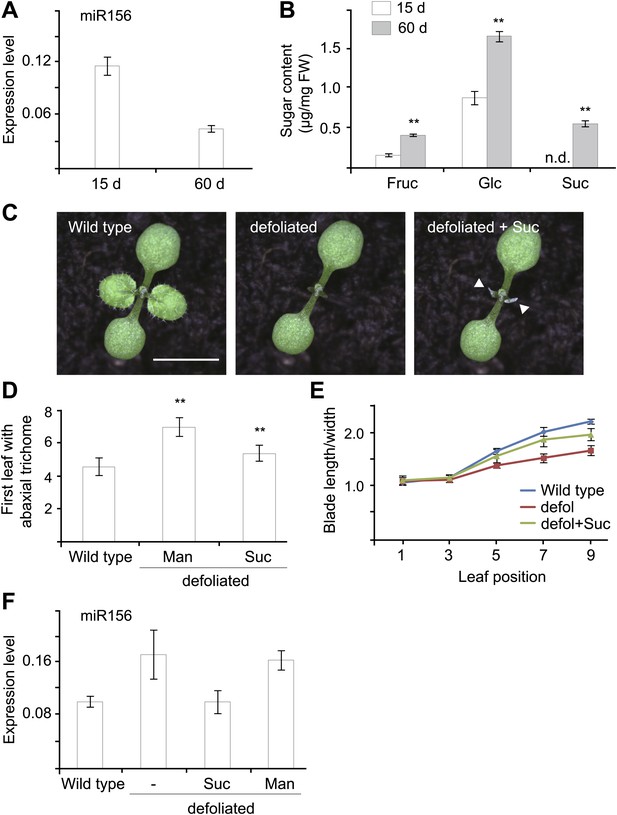
Sugar as a mobile signal to trigger vegetative phase transition.
(A) Expression of miR156 in 15-day-old and 60-day-old wild type plants grown under short day conditions. (B) Sugar measurement. Fifteen-day-old and 60-day-old short day plants were collected at Zeitgeber time 16. The fructose (Fru), glucose (Glc), and sucrose (Suc) content was analyzed by GC-MS and quantified. **Significant difference from 15-day-old wild type plants, Student t-test, p<0.001. Error bars indicate SD. n.d.: undetected; FW: fresh weight. (C) Seven-day-old wild type Arabidopsis seedlings before and after defoliation. Arrows indicate where the lanolin-sucrose (Suc) paste was applied. Scale bar indicates 0.5 cm. (D and E) Seven-day-old wild type seedlings before and after defoliation. Appearance of the first abaxial trichome (D) and the length-to-width ratios of blades (E) were measured. n=10. **Significant difference from wild type, Student t-test, p<0.001. Error bars indicate SE. defol: defoliated; Suc: sucrose. (F) Expression of miR156. Seven-day-old wild type seedlings were defoliated and sucrose (Suc) or mannitol (Man) was applied to the defoliated petioles. The shoot apices were collected for expression analyses 2 days after defoliation.
We then performed defoliation assays. The blades of the first two leaves of 7-day-old wild type seedlings were manually removed. Then 50 mM sucrose or mannitol (as control) was applied to the petioles of the defoliated leaves (Figure 4C). Consistent with the previous report (Yang et al. 2011), the removal of the first two leaves resulted in an increased level of miR156 in the shoot apices (Figure 4F). The expression of adult-specific traits was accordingly delayed. Compared to intact plants, the production of abaxial trichomes in the defoliated plants was delayed by 1.0 plastochrons (Figure 4D), and the increase in the length-to-width ratio of the lamina was slower (Figure 4E).
Sucrose application partially suppressed the delay in the juvenile-to-adult phase transition caused by defoliation. The sucrose-treated plants produced the abaxial trichomes 0.8 plastochrons later than intact wild type plants, but 1.6 plastochrons earlier than the mannitol-treated plants (Figure 4D). In addition, the length-to-width ratios of the fifth, seventh, and ninth leaves in the sucrose-treated plants were higher than those in the mannitol-treated plants (Figure 4E). In agreement with these phenotypic differences, the expression of miR156 was reduced in the apices of the sucrose-treated plants but not in those treated with mannitol (Figure 4F).
A reduced photosynthetic rate delays the juvenile-to-adult phase transition
To confirm the role of sugar in the juvenile-to-adult phase transition, we analyzed the Arabidopsis cao/chlorina1 (ch1) mutant. A mutation in CAO/CH1 (At1g44446), which encodes chlorophyll (Chl) a oxygenase, causes a reduced level of Chl b and low efficiency of photosynthesis (Espineda et al., 1999). Compared to the wild type, the cao/ch1 mutant developed smaller pale green leaves and had a prolonged juvenile phase (Figure 5—figure supplement 1). The rosette leaves in the cao/ch1 mutant were rounder than those in the wild type plant (Figure 5A,B). Additionally, the appearance of abaxial trichomes in the cao/ch1 mutant was delayed (Figure 5C). Expression analyses indicated that higher levels of miR156 accumulated in the cao/ch1 mutant than in the wild type plant (Figure 5D).
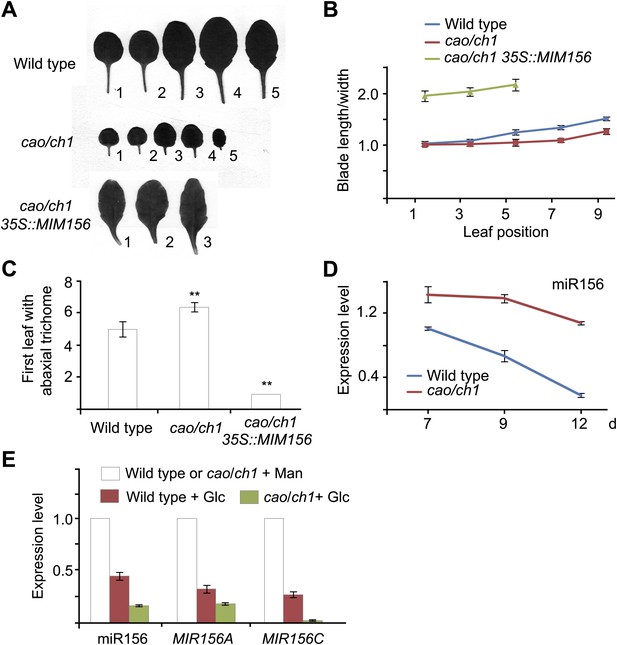
cao/ch1 mutant impairs vegetative phase transition.
(A) Leaf morphology of wild type, cao/ch1, and 35S::MIM156 cao/ch1 plants under long day conditions. The leaves from 15-day-old plants were detached and scanned. The numbers indicate leaf positions. (B and C) The length-to-width ratio of the blade (B) and the appearance of the first abaxial trichome (C). n=12. (D) Expression of miR156 during development. Wild type plants and cao/ch1 mutants were collected at 7, 9, or 12 days after germination under long day conditions. (E) Expression of miR156, pri-MIR156A, and pri-MIR156C. Five-day-old wild type and cao/ch1 mutants in 1/2 Murashige and Skoog (MS) liquid media were treated with 50 mM glucose or mannitol for 1 day. The expression levels in the mannitol-treated wild type or cao/ch1 were set to 1. The treatment was started at Zeitgeber time 12. **Significant difference from wild type, Student t-test, p<0.001. Error bars indicate SE.
To examine whether the delayed phase transition in cao/ch1 depends on miR156 function, we crossed 35S::MIM156 into cao/ch1. Similarly to 35S::MIM156, 35S::MIM156 cao/ch1 produced the abaxial trichomes on the first leaf, and the leaves were elongated and serrated (Figure 5A–C; Figure 5—figure supplement 1). Compared to the wild type, the cao/ch1 mutants exhibited higher glucose sensitivity. Treatment of cao/ch1 seedlings with 50 mM glucose significantly reduced the level of miR156 (Figure 5E). Taken together, we conclude that sugar from the pre-existing leaves acts as a mobile signal to trigger the juvenile-to-adult phase transition through repression of miR156 in the young leaf primordia.
Repression of miR156 by sugar is evolutionarily conserved
miR156 is present in all major plant taxa (Axtell and Bowman, 2008). To test whether the regulation of miR156 by sugar is evolutionarily conserved, we examined the expression of miR156 in response to sugar in other plants, including Nicotiana benthamiana (tobacco), Physcomitrella patens (moss), and Solanum lycopersicum (tomato).
N. benthamiana and S. lycopersicum were grown in 1/2 MS liquid media without sugar. After the first two leaves appeared, the seedlings were treated with 50 mM sucrose for 2 days. The seedlings of N. benthamiana and S. lycopersicum were collected and used for expression analyses. For P. patens, the sugar treatment was conducted during the protonema stage. Compared to those treated with mannitol, the amount of miR156 was greatly reduced in all the sucrose-treated plants (Figure 6), indicating that repression of miR156 by sugar is evolutionarily conserved.
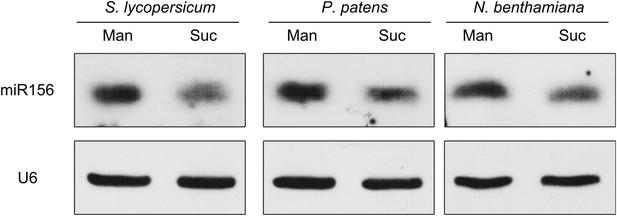
Repression of miR156 by sugar is evolutionarily conserved.
Expression of miR156 in Physcomitrella patens, Solanum lycopersicum, and Nicotiana benthamiana. The plants were treated with 50 mM sucrose (Suc) or mannitol (Man) for 2 days. U6 was monitored as the loading control. Treatment was started at Zeitgeber time 12.
Sugar regulates miR156 expression at both the transcriptional and post-transcriptional level
To investigate at which level sugar represses miR156, we performed chromatin immunoprecipitation analyses (ChIP) using anti-RNA polymerase II (anti-Pol II) antibody, which recognizes the C-terminal heptapeptide repeat of RNA Pol II and has been used to correlate RNA Pol II binding with gene expression. Enrichment of the promoter fragments of MIR156A and MIR156C was compared between the seedlings treated with mannitol and those treated with glucose. As shown in Figure 7A, the promoter fragments (harboring TATA boxes) of MIR156A and MIR156C were substantially enriched in the mannitol-treated seedlings, but not in those treated with glucose, indicating that glucose induces transcriptional repression of MIR156A and MIR156C (Figure 7A).
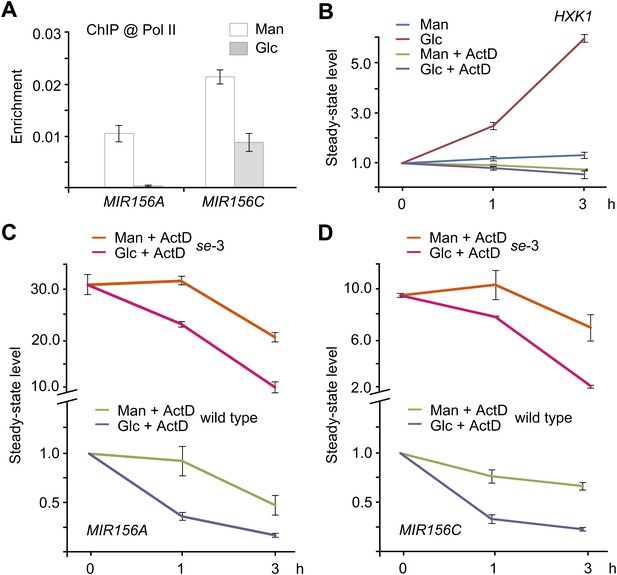
Sugar promotes the degradation of miR156 primary transcripts.
(A) Chromatin immunoprecipitation (ChIP) analyses. Five-day-old wild type seedlings were treated with 50 mM glucose (Glc) or mannitol (Man) for 1 day. Anti-Pol II was used for ChIP analyses. The genomic fragments near the MIR156A or MIR156C TATA box were amplified. Relative enrichment was calculated by the ratio of bound DNAs after ChIP to input DNAs. (B) Expression of HXK1 in response to glucose. Five-day-old wild type seedlings in 1/2 Murashige and Skoog (MS) liquid media were pre-treated with or without actinomycin (ActD) for 12 hr. The seedlings were harvested at 0, 1, and 3 hr after 50 mM glucose or mannitol was added. The expression level at 0 hr was set to 1. (C and D) Expression of pri-MIR156A (C) and pri-MIR156C (D) in the wild type and se-3 mutant. Five-day-old wild type seedlings in 1/2 MS liquid media were pre-treated with ActD for 12 hr. The seedlings were then treated with 50 mM glucose or mannitol. The expression levels of pri-MIR156A and pri-MIR156C in the wild type at 0 hr were set to 1. Sugar treatment was started at Zeitgeber time 12.
We next examined the effect of actinomycin-D (ActD), which blocks transcription. To test transcription blocking efficiency, we analyzed the expression of HXK1, which is rapidly induced by glucose (Price et al., 2004). The transcript level of HXK1 was increased about fourfold after 3 h of glucose treatment. By contrast, the expression of HXK1 was not altered in the seedlings treated with glucose and ActD (Figure 7B).
The addition of ActD did not affect repression of pri-MIR156C by glucose. The transcript level of pri-MIR156C was reduced by about 75% after 3 hr in the presence of glucose, compared to a 30% reduction in the presence of mannitol (Figure 7D). A similar expression pattern was observed in pri-MIR156A (Figure 7C), suggesting that glucose modulates miR156 expression at the post-transcriptional level through the degradation of pri-MIR156.
To investigate whether the reduction in miR156 primary transcripts after glucose treatment was caused by an increase in the processing efficiency of pri-MIR156, we performed the glucose treatment assay using the serrate (se) mutant which is defective in miRNA biogenesis (Grigg et al., 2005; Lobbes et al., 2006; Yang et al., 2006; Laubinger et al., 2008). Similar to the wild type, the amount of pri-MIR156A and pri-MIR156C was markedly decreased in the ActD/glucose-treated se-3 mutant (Figure 7C,D), indicating that glucose regulates the abundance of pri-MIR156 independently of the miRNA processing machinery.
HXK1 encodes a glucose sensor that transduces diverse sugar signals. gin2-1, the HXK1-null mutant (Moore et al. 2003), exhibited a lower level of miR156 than the wild type (Figure 8A). The expression of miR156 still decreased over time in the gin2-1 mutant (Figure 8B). To test whether the repression of miR156 by sugar is mediated by HXK1, we compared the glucose response between the wild type and the gin2-1 mutant. The expression of pri-MIR156A and pri-MIR156C was reduced after sugar treatment in both the wild type and the gin2-1 mutant (Figure 8C,D). Similarly, an evident decrease in pri-MIR156C was observed in the gin2-1 seedlings treated with ActD/glucose (Figure 8E). These results suggest that HXK1 plays a role in miR156 expression but is not absolutely required for the repression of miR156 by sugar.
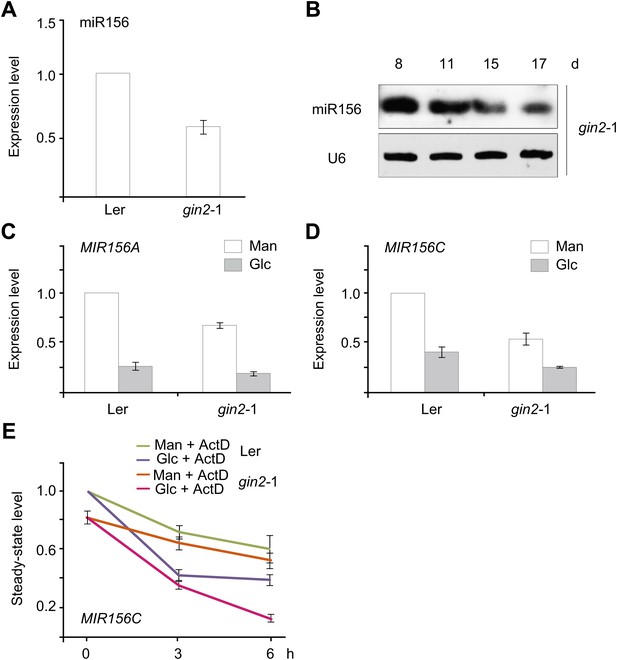
The role of HXK1 in sugar-induced miR156 repression.
(A) Expression of miR156 in the 5-day-old wild type (ecotype Ler) and gin2-1 mutant. The expression level of miR156 in Ler was set to 1. (B) Time course analyses of miR156 in the gin2-1 mutant. (C and D) Expression of pri-MIR156A (C) and pri-MIR156C (D) in response to glucose in the wild type (ecotype Ler) and gin2-1 mutant. Five-day-old seedlings in 1/2 Murashige and Skoog (MS) liquid media were treated with 50 mM glucose (Glc) or mannitol (Man) for 6 hr. The expression level in Ler at 0 h was set to 1. (E) Expression of pri-MIR156C in Ler and gin2-1. Five-day-old seedlings in 1/2 MS liquid media were pre-treated with actinomycin-D (ActD) for 12 hr and then treated with 50 mM glucose or mannitol. The expression level of pri-MIR156C in Ler at 0 hr was set to 1. Sugar treatment was started at Zeitgeber time 12.
We then performed the sugar treatment assay in the presence of ActD and cycloheximide (CHX), an inhibitor of protein synthesis. The level of pri-MIR156C transcripts was greatly reduced in the ActD-treated samples, but not in those treated with both ActD and CHX (Figure 7—figure supplement 1), suggesting that sugar-induced pri-MIR156C degradation requires de novo protein synthesis.
mRNAs can be degraded through several partially independent pathways, including nonsense-mediated mRNA decay (NMD), 5′-to-3′ mRNA degradation via exonucleases, and 3′-to-5′ mRNA degradation via the exosome. The UP-frameshift (UPF) proteins, UPF1, UPF2, and UPF3, are essential for the NMD function in plants (Arciga-Reyes et al., 2006; Kurihara et al., 2009). It has been shown that upf1 and upf3 mutants impair the sugar response and over-accumulate sugar-inducible mRNAs (Yoine et al. 2006). Therefore, we investigated the role of UPF in the sugar-mediated repression of miR156. Compared to the wild type, the expression of pri-MIR156A and pri-MIR156C was slightly increased in upf1-5 and upf3-1 mutants (Figure 7—figure supplement 2A). Glucose was still able to repress the accumulation of pri-MIR156C, albeit to a lesser extent (Figure 7—figure supplement 2B), indicating that sugar promotes pri-MIR156 degradation independently of canonical NMD.
Discussion
Sugar as an endogenous timer for the juvenile-to-adult phase transition
Based on expression analyses, defoliation experiments, and photosynthetic mutant characterization, we show that sugar acts upstream of miR156. We propose a model explaining how sugar regulates the juvenile-to-adult phase transition through modulation of miR156 expression as follows. After seed germination, plants start accumulating sugars through photosynthesis. Sucrose, the major transportable sugar, moves from the pre-existing leaves to the young leaf primordia, where its hydrolytic hexose product, glucose, represses the expression of miR156. As a result, the level of SPL increases and the expression of adult traits is promoted.
Identification of sugar as the endogenous developmental timing cue explains the irreversible nature of the age pathway. The level of miR156 is destined to decrease because the gradual accumulation of carbohydrates is inevitable and essential for plant growth and development. In Caenorhabditis elegans, the transitions between the stages of larval development are controlled by the sequential action of two miRNAs, lin-4 and let-7 (Pasquinelli and Ruvkun, 2002; Moss, 2007; Ambros, 2011). In contrast to miR156, the expression of these two miRNAs is increased with age. It will be intriguing to examine whether sugar/carbohydrates or nutrients from the diet triggers the upregulation of lin-4 and let-7 in worms.
Cellular carbon (C) and nitrogen (N) are tightly coordinated to sustain optimal plant growth (Raven et al., 2004; Zheng, 2009). C compounds including many carbohydrates such as sucrose and glucose are synthesized in the leaf, while N nutrients such as nitrate and ammonium are assimilated by the root system. Biochemical and physiological studies have demonstrated long-distance sensing and signaling of the C/N balance in plants. When soil is short of and , photosynthesis in the leaf is inhibited. Whether miR156 and the juvenile-to-adult transition respond to N excess or deficient conditions is another interesting topic awaiting investigation.
Does sugar promote flowering through miR156?
In addition to the juvenile-to-adult transition, flowering is of great importance for reproductive success in plants. Previous studies revealed that the floral transition is regulated by diverse environmental factors, such as photoperiod, temperature, and light, in combination with the endogenous signal derived from nutritional status. The nutrient-dependent regulation of flowering is likely dependant on the rate of sucrose export from source leaves (Corbesier et al., 1998; Sivitz et al., 2007). This notion is supported by our observations that sugar from pre-existing leaves acts as a long-distance signal to repress the expression of miR156 in young leaf primordia, and that a high level of miR156 delays flowering (Wang et al., 2009). Intriguingly, a recent study has demonstrated that INDETERMINATE DOMAIN transcription factor AtIDD8 regulates photoperiodic flowering by modulating sugar transport and metabolism (Seo et al., 2011), suggesting that additional sugar-mediated flowering pathways exist.
Sugar, produced in mesophyll cells in leaves, is transported from source tissues to sink tissues through vascular bundles (Kuhn and Grof, 2010; Ayre, 2011). In Arabidopsis, sucrose transporters are involved in loading sucrose into the phloem in source leaves and the uptake of sucrose into the cells of sink tissues such as roots, fruit, and developing leaves (Williams et al., 2000). Very recently, the sucrose effluxers, SWEET11 and SWEET12, which facilitate sucrose efflux into the cell wall of companion cells, have been identified (Chen et al., 2011). It is therefore interesting to investigate whether impairment of sucrose transport from leaf cells into the vascular system causes a defect in miR156 expression and developmental transitions.
Regulation of miR156 by sugar in a complex manner
There are several means by which sugar regulates gene expression. For example, sugar decreases the transcript level of rice AMY3 at both the transcriptional and post-transcriptional level. It was shown that destabilization of the mRNAs of AMY3 is mediated by its 3′ untranslated region (UTR) (Chan and Yu, 1998). Similarly, we found that pri-MIR156A and pri-MIR156C are subjected to transcriptional repression as well as transcript degradation in response to glucose. This two-level expression control by sugar might contribute to robust repression of miR156, which leads to irreversible transition from the juvenile to adult phase in plants. In Arabidopsis, HXK1 is a glucose sensor that transduces diverse aspects of sugar response. For example, the gin2-1 mutant reduces shoot and root growth, delays flowering, increases apical dominance, and alters sensitivity to auxin and cytokinin (Moore et al., 2003). However, we did not observe an obvious juvenile-to-adult phase phenotype in the gin2-1 mutant under long day conditions (data not shown). Further studies will determine if the transcriptional repression of miR156 by sugar is mediated by the previously identified nuclear-localized HXK1-VHA-B1-RPT5B complex.
The level of miR156 is greatly reduced when plants are treated with both glucose and sucrose. Since these sugars can be easily interconverted, it remains unclear whether the repression of miR156 is hexose or sucrose-dependent. Moreover, based on pharmacological treatment and mutant analyses, we show that sugar is able to trigger the degradation of pri-MIR156A/C independently of the canonical glucose sensor, HXK1. Thus, investigation of the molecular mechanism by which sugar in particular recognizes pri-MIR156 and promotes their degradation is an important goal for future research.
Materials and methods
Plant materials
Request a detailed protocolA. thaliana, P. patens, S. lycopersicum, and N. benthamiana were grown at 21°C (day)/19°C (night) under long day (16 hr light/8 hr dark) or short day (8 hr light/16 hr dark) conditions. White light was provided by a 4:2 mixture of cool white fluorescent lamps (Lifemax cool daylight 36W/865; Philips Lighting Co., Shangai, China) and warm white fluorescent lamps (Lifemax warm white 36W/830; Philips Lighting Co.). Light intensity was 80 µmol/m2/s in long day and 90 µmol/m2/s in short day conditions. mir156a (SALK_056809), mir156c (SALK_004679), cao/ch1, and gin2-1 mutants were ordered from the Arabidopsis Biological Resource Center (Columbus, OH). 35S::MIM156 was described (Wang et al., 2008).
Plant treatment
Request a detailed protocolAll treatment assays were carried out under long day conditions. Defoliation assays were performed as described (Yang et al., 2011). For the sugar treatment assay, Arabidopsis seeds were sterilized with 20% bleach and germinated in 50 ml 1/2 MS liquid media with shaking at 140 rpm. The seedlings were then transferred to 1/2 MS media supplemented with sugar. For the sugar starvation assay, 5-day-old wild type seedlings grown in 1/2 MS liquid media supplemented with 50 mM sucrose were transferred to 1/2 MS liquid media free of sugar and grown in the dark for 2 days. For the ActD and CHX assay, 20 µg/ml ActD (Sigma-Aldrich, Beijing, China) or 100 µM CHX (Sigma-Aldrich) was used. P. patens was cultured as described (Cove et al., 2009). The plants in the protonema stage were used for the sugar treatment assay. Seedlings of S. lycopersicum and N. benthamiana were treated with 50 mM sucrose for 2 days.
Expression analyses
Request a detailed protocolTotal RNA was extracted with Trizol reagent (Invitrogen, Life Technologies, Shanghai, China). Then 1 µg of total RNA was DNase I-treated and used for cDNA synthesis with an oligo (dT) primer. The qRT-PCR primers for SPL3, SPL9, SPL15, and TUB have been described (Wang et al., 2008; Wang et al., 2009). The primer sequences for other genes are shown in supplementary file 1B. A small RNA blot was performed as described (Wang et al., 2009). qRT-PCR on mature miR156 was performed according to a published protocol (Varkonyi-Gasic et al., 2007).
ChIP analyses
Request a detailed protocolChIP analysis was performed according to protocol (Wang et al., 2009). Crude chromatin extract was pulled down with anti-Pol II antibodies (Abcam, Hong Kong, China). ChIP DNAs were reverse crosslinked and purified using a PCR purification kit (Qiagen, Shanghai, China). A 1 μl sample of DNA was used for real-time PCR analyses. The relative enrichment of each fragment was calculated by the ratio of bound DNAs after ChIP to input DNAs.
Sugar measurement
Request a detailed protocolWild type plants were grown under short day conditions. Then 15-day-old juvenile or 50-day-old adult plants were collected at ZT 16. Sugar was measured using 50 mg (fresh weight) of tissue. Sample extraction, preparation, and analyses were performed as previously described (Tan et al., 2011). The individual sugar was identified based on the retention time and mass spectrometry standards. Quantification was performed by an external standard method.
References
-
MicroRNAs and developmental timingCurr Opin Genet Dev 21:511–517.https://doi.org/10.1016/j.gde.2011.04.003
-
Evolution of plant microRNAs and their targetsTrends Plant Sci 13:343–349.https://doi.org/10.1016/j.tplants.2008.03.009
-
The 3' untranslated region of a rice alpha-amylase gene functions as a sugar-dependent mRNA stability determinantProc Natl Acad Sci USA 95:6543–6547.
-
The role of carbohydrates in the induction of flowering in Arabidopsis thaliana: comparison between the wild type and a starchless mutantPlanta 206:131–137.
-
Culturing the moss Physcomitrella patensCold Spring Harb Protoc, db prot5136, 10.1101/pdb.prot5136.
-
The AtCAO gene, encoding chlorophyll a oxygenase, is required for chlorophyll b synthesis in Arabidopsis thalianaProc Natl Acad Sci USA 96:10507–10511.
-
Sucrose transporters of higher plantsCurr Opin Plant Biol 13:288–298.https://doi.org/10.1016/j.pbi.2010.02.001
-
Genome-wide suppression of aberrant mRNA-like noncoding RNAs by NMD in ArabidopsisProc Natl Acad Sci USA 106:2453–2458.https://doi.org/10.1073/pnas.0808902106
-
Control of developmental timing by micrornas and their targetsAnnu Rev Cell Dev Biol 18:495–513.https://doi.org/10.1146/annurev.cellbio.18.012502.105832
-
The past, present, and future of vegetative phase changePlant Physiol 154:541–544.https://doi.org/10.1104/pp.110.161620
-
Sugar sensing and signalling networks in plantsBiochem Soc Trans 33:269–271.https://doi.org/10.1042/BST0330269
-
FLAGdb/FST: a database of mapped flanking insertion sites (FSTs) of Arabidopsis thaliana T-DNA transformantsNucleic Acids Res 30:94–97.
-
Arabidopsis sucrose transporter AtSUC9. High-affinity transport activity, intragenic control of expression, and early flowering mutant phenotypePlant Physiol 143:188–198.
-
Sugar signals and molecular networks controlling plant growthCurr Opin Plant Biol 13:274–279.https://doi.org/10.1016/j.pbi.2009.12.002
-
Sugar transporters in higher plants–a diversity of roles and complex regulationTrends Plant Sci 5:283–290.
-
The role of hexokinase in plant sugar signal transduction and growth and developmentPlant Mol Biol 44:451–461.
-
The lba1 mutation of UPF1 RNA helicase involved in nonsense-mediated mRNA decay causes pleiotropic phenotypic changes and altered sugar signalling in ArabidopsisPlant J 47:49–62.
-
Carbon and nitrogen nutrient balance signaling in plantsPlant Signal Behav 4:584–591.
Article and author information
Author details
Funding
National Natural Science Foundation of China (31222029; 91217306)
- Jia-Wei Wang
State Key Basic Research Program of China (2013CB127000)
- Jirong Huang
- Jia-Wei Wang
Recruitment Program of Global Expects (China)
- Jia-Wei Wang
Shanghai Pujiang Program (12PJ1409900)
- Jia-Wei Wang
Initiation grant from National Key Laboratory of Plant Molecular Genetics (Institute of Plant Physiology and Ecology, Shanghai Institutes for Biological Sciences)
- Jia-Wei Wang
Chinese Academy of Sciences (KSCX2-YW-N-069)
- Guodong Wang
The funders had no role in study design, data collection and interpretation, or the decision to submit the work for publication.
Acknowledgements
We thank Li Yang and Scott Poethig for the provision of unpublished data; Hong-Tao Liu for discussion; Han Xiao for S. lycopersicum seeds; and the Arabidopsis Biological Resource Center for cao/ch1, gin2-1, and MIR156 T-DNA insertion mutants.
Copyright
© 2013, Yu et al.
This article is distributed under the terms of the Creative Commons Attribution License, which permits unrestricted use and redistribution provided that the original author and source are credited.
Metrics
-
- 4,337
- views
-
- 1,098
- downloads
-
- 262
- citations
Views, downloads and citations are aggregated across all versions of this paper published by eLife.
Download links
Downloads (link to download the article as PDF)
Open citations (links to open the citations from this article in various online reference manager services)
Cite this article (links to download the citations from this article in formats compatible with various reference manager tools)
Further reading
-
- Developmental Biology
- Plant Biology
By regulating the expression of key microRNA molecules, sugar levels in leaves control the transition from the juvenile to the adult form in plants.
-
- Plant Biology
It is well documented that type-III effectors are required by Gram-negative pathogens to directly target different host cellular pathways to promote bacterial infection. However, in the context of legume–rhizobium symbiosis, the role of rhizobial effectors in regulating plant symbiotic pathways remains largely unexplored. Here, we show that NopT, a YopT-type cysteine protease of Sinorhizobium fredii NGR234 directly targets the plant’s symbiotic signaling pathway by associating with two Nod factor receptors (NFR1 and NFR5 of Lotus japonicus). NopT inhibits cell death triggered by co-expression of NFR1/NFR5 in Nicotiana benthamiana. Full-length NopT physically interacts with NFR1 and NFR5. NopT proteolytically cleaves NFR5 both in vitro and in vivo, but can be inactivated by NFR1 as a result of phosphorylation. NopT plays an essential role in mediating rhizobial infection in L. japonicus. Autocleaved NopT retains the ability to cleave NFR5 but no longer interacts with NFR1. Interestingly, genomes of certain Sinorhizobium species only harbor nopT genes encoding truncated proteins without the autocleavage site. These results reveal an intricate interplay between rhizobia and legumes, in which a rhizobial effector protease targets NFR5 to suppress symbiotic signaling. NFR1 appears to counteract this process by phosphorylating the effector. This discovery highlights the role of a bacterial effector in regulating a signaling pathway in plants and opens up the perspective of developing kinase-interacting proteases to fine-tune cellular signaling processes in general.