DNA Helicases: Preparing to unwind
Life begets life: in order to propagate itself, a living organism must replicate its genetic material and pass this on to the next generation. To duplicate a molecule of double-stranded DNA within a cell, an ‘initiation factor’ recognises a stretch of DNA called an origin of replication and then recruits an enzyme called a helicase that goes on to unwind the double helix. This process exposes the two strands of DNA and allows them to act as templates to guide the synthesis of two new strands of DNA—which are called the leading and lagging strands. The end result is two identical molecules of double-stranded DNA.
All helicases involved in DNA replication are shaped like a ring made of six subunits and they work by selecting, encircling and sliding (or translocating) along a single strand of DNA, while keeping the other strand on the outside (Soultanas, 2012). Now, in eLife, researchers at St. Jude Children’s Research Hospital and the Massachusetts Institute of Technology (MIT) show how the leading strand template becomes selected as the ‘translocation strand’ by a helicase at a molecular level (Froelich et al., 2014).
Although DNA replication is vital to living things, the mechanisms behind the steps in this process are far from conserved in the different domains of life. In bacteria, for example, two copies of the helicase are loaded as single rings onto an already separated DNA duplex, and they immediately start moving in opposite directions along the lagging strands to unwind the DNA (Mott and Berger, 2007).
In eukaryotes, on the other hand, things happen more slowly. The helicase complex—called ‘Minichromosome Maintenance 2-7 complex’ or Mcm2-7 for short—also forms a ring made of six subunits (Figure 1A). However, two complexes are loaded onto the DNA as a double ring, and the rings only start moving along the leading strand templates after multiple chemical modifications have been made and various other activating factors have been recruited (Remus and Diffley, 2009; Remus et al., 2009; Fu et al., 2011).
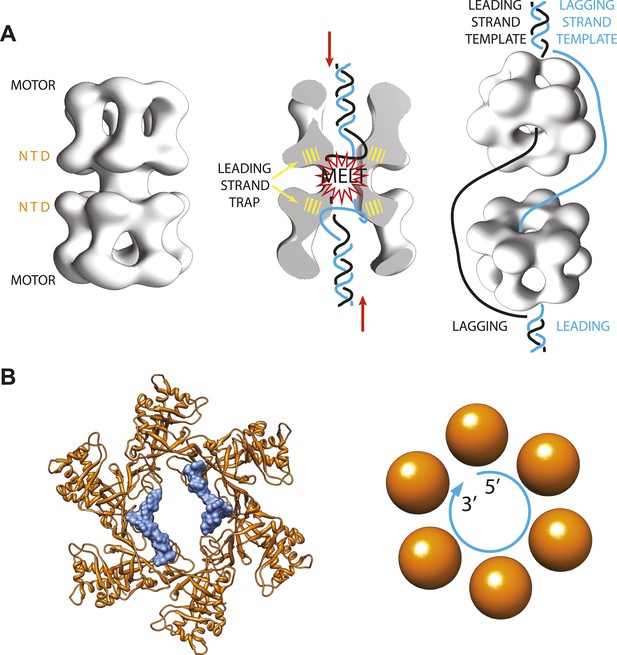
Archaeal/eukaryotic helicase interacting with DNA.
(A) DNA opening and unwinding by the Mcm2-7 helicase: the two motor domains that move along the DNA are at opposite ends of a DNA-loaded helicase double ring, with the N-terminal DNA-binding domains (NTD) in the middle. Froelich, Kang et al. propose that the motor domains push the duplex DNA towards the middle of the helicase, hence promoting melting of the DNA at the origin of replication and trapping of the leading strand template by the NTD. The rings then separate and travel in opposite directions, with one ring sliding along each of the leading strand templates from the two replication forks. (B) Crystal structure of the MCM helicase (orange) bound to single-stranded DNA (light blue). The DNA circles around the MCM central channel in a clockwise direction (when travelling from the 5′-end to 3′-end and viewing the ring from its C-terminal face).
Three events must occur in order to activate the origin of replication in eukaryotes: the two Mcm2-7 rings must separate; each ring must trap the leading strand template; and each ring must eject the lagging strand template. However, we know very little about the details of any of these three steps in eukaryotes. And we know even less about them in archaea, although we do know that copies of proteins involved in DNA replication found in this domain of life are related to those found in eukaryotes.
Eric Enemark of St. Jude, Stephen Bell of MIT and co-workers—including Clifford Froelich and Sukhyun Kang as joint first authors—have used a combination of protein crystallography and biochemistry to shed light on the process that activates the origin of replication (Froelich et al., 2014). The Enemark lab determined the three-dimensional structure of a single-stranded fragment of DNA bound to a helicase ring from a species of archaea that lives in deep ocean vents. This helicase, called MCM, is the archaeal equivalent of the eukaryotic Mcm2-7 complex, and is easier to study because it is made of six identical protein subunits, whereas Mcm2-7 is made of six different protein subunits. Nevertheless, the two complexes are thought to be similar enough that general conclusions might be translated from one complex to the other.
As expected, the DNA binds inside the centre of the MCM ring, but it follows a different path from the DNA seen in previous helicase-DNA complex structures (Enemark and Joshua-Tor, 2006; Itsathitphaisarn et al., 2012). In fact, in the new MCM structure solved by the Enemark lab the DNA does not run straight through the ring channel but rather circles around its interior (Figure 1B). Closer inspection suggests that the N-terminal DNA-binding domain of the MCM helicase—which is the only portion of the protein present in the crystal structure—can only trap the leading (rather than lagging) strand template. This is also in agreement with previously published studies (McGeoch et al., 2005; Barry et al., 2007; Bochman and Schwacha, 2008).
Interestingly, the diameter of the MCM-bound DNA circle is much larger than that of a DNA double helix, and the points of contact between the DNA and the proteins involve sites that are usually protected in the duplex form of DNA. This suggests that the new MCM-DNA crystal structure reported by Froelich, Kang et al. might have captured an intermediate form of DNA that exists when the origin of replication melts at the onset of DNA replication (Figure 1A). This notion is also compatible with the results of in vitro experiments with related proteins from yeast, which were conducted in the Bell lab at MIT (Froelich et al. 2014).
The MCM residues that contact the DNA in the archaeal structure are fully conserved only in three of the six subunits of the eukaryotic protein complex. Remarkably, all these residues are close to one another and constitute an extended single-stranded-binding surface on the Mcm2-7 that is proposed to function in DNA melting and strand selection. A combined mutant of these three subunits can, in fact, still be loaded onto double-stranded DNA, albeit with low efficiency. However, the mutant cannot bind single stranded DNA, does not recruit some of the factors that are essential for origin activation, and it cannot support DNA replication and cell division.
Altogether, this study provides new insights into the mechanisms that activate the origin of replication in eukaryotes by identifying a role for the Mcm2-7 helicase in the processes of strand selection and, probably, origin melting. Froelich, Kang et al. propose that the two Mcm2-7 motor domains must play an important function in the early stages of origin activation, pumping duplex DNA towards the centre of the complex, and hence promoting duplex melting and the subsequent capture of the leading strand by the helicase. Determining how the Mcm2-7 motor domain engages with DNA is an important challenge for future studies.
References
-
Archaeal MCM has separable processivity, substrate choice and helicase domainsNucleic Acids Research 35:988–998.https://doi.org/10.1093/nar/gkl1117
-
The Mcm2-7 complex has in vitro helicase activityMolecular Cell 31:287–293.https://doi.org/10.1016/j.molcel.2008.05.020
-
Organization of the archaeal MCM complex on DNA and implications for the helicase mechanismNature Structural & Molecular Biology 12:756–762.https://doi.org/10.1038/nsmb974
-
DNA replication initiation: mechanisms and regulation in bacteriaNature Reviews Microbiology 5:343–354.https://doi.org/10.1038/nrmicro1640
-
Eukaryotic DNA replication control: lock and load, then fireCurrent Opinion in Cell Biology 21:771–777.https://doi.org/10.1016/j.ceb.2009.08.002
-
Loading mechanisms of ring helicases at replication originsMolecular microbiology 84:6–16.https://doi.org/10.1111/j.1365-2958.2012.08012.x
Article and author information
Author details
Publication history
Copyright
© 2014, Zhou and Costa
This article is distributed under the terms of the Creative Commons Attribution License, which permits unrestricted use and redistribution provided that the original author and source are credited.
Metrics
-
- 1,147
- views
-
- 58
- downloads
-
- 1
- citations
Views, downloads and citations are aggregated across all versions of this paper published by eLife.
Download links
Downloads (link to download the article as PDF)
Open citations (links to open the citations from this article in various online reference manager services)
Cite this article (links to download the citations from this article in formats compatible with various reference manager tools)
Further reading
-
- Biochemistry and Chemical Biology
Identifying target proteins for bioactive molecules is essential for understanding their mechanisms, developing improved derivatives, and minimizing off-target effects. Despite advances in target identification (target-ID) technologies, significant challenges remain, impeding drug development. Most target-ID methods use cell lysates, but maintaining an intact cellular context is vital for capturing specific drug–protein interactions, such as those with transient protein complexes and membrane-associated proteins. To address these limitations, we developed POST-IT (Pup-On-target for Small molecule Target Identification Technology), a non-diffusive proximity tagging system for live cells, orthogonal to the eukaryotic system. POST-IT utilizes an engineered fusion of proteasomal accessory factor A and HaloTag to transfer Pup to proximal proteins upon directly binding to the small molecule. After significant optimization to eliminate self-pupylation and polypupylation, minimize depupylation, and optimize chemical linkers, POST-IT successfully identified known targets and discovered a new binder, SEPHS2, for dasatinib, and VPS37C as a new target for hydroxychloroquine, enhancing our understanding these drugs’ mechanisms of action. Furthermore, we demonstrated the application of POST-IT in live zebrafish embryos, highlighting its potential for broad biological research and drug development.
-
- Biochemistry and Chemical Biology
- Structural Biology and Molecular Biophysics
Inhibition of Bruton’s tyrosine kinase (BTK) has proven to be highly effective in the treatment of B-cell malignancies such as chronic lymphocytic leukemia (CLL), autoimmune disorders, and multiple sclerosis. Since the approval of the first BTK inhibitor (BTKi), Ibrutinib, several other inhibitors including Acalabrutinib, Zanubrutinib, Tirabrutinib, and Pirtobrutinib have been clinically approved. All are covalent active site inhibitors, with the exception of the reversible active site inhibitor Pirtobrutinib. The large number of available inhibitors for the BTK target creates challenges in choosing the most appropriate BTKi for treatment. Side-by-side comparisons in CLL have shown that different inhibitors may differ in their treatment efficacy. Moreover, the nature of the resistance mutations that arise in patients appears to depend on the specific BTKi administered. We have previously shown that Ibrutinib binding to the kinase active site causes unanticipated long-range effects on the global conformation of BTK (Joseph et al., 2020). Here, we show that binding of each of the five approved BTKi to the kinase active site brings about distinct allosteric changes that alter the conformational equilibrium of full-length BTK. Additionally, we provide an explanation for the resistance mutation bias observed in CLL patients treated with different BTKi and characterize the mechanism of action of two common resistance mutations: BTK T474I and L528W.