Pancreatic Cancer: Change isn't always better
Pancreatic cancer is the fourth most common cause of cancer-related death in the United States, with only 6% of people surviving for five years after diagnosis. Now, in eLife, Charles Murtaugh and co-workers – including Nathan Krah as first author – shed new light on the process that leads normal pancreatic cells to become cancer cells (Krah et al., 2015).
The exocrine pancreas is the part of the pancreas that produces the enzymes that help to digest food, and is also the region where pancreatic cancer arises. It remains unclear how normal exocrine cells – two types of which are the acinar and ductal cells – become cancerous. Pancreatic cancer – as well as the pre-cancerous lesions that precede it – consists of cells that resemble ductal cells. It had thus been assumed that ductal cells are the origin of this disease. However, this notion has been challenged by recent experimental evidence from genetically engineered mice that suggests that acinar cells are in fact the most common source of pancreatic cancer (for review see Puri et al., 2015).
For acinar cells to give rise to cancer, they first must lose their acinar characteristics and become duct-like, in a process known as acinar-to-ductal metaplasia (or ADM). Then, these duct-like cells transform into cancer precursor cells and, in time, become cancer cells. This cancerous transformation is associated with the presence of a form of the Kras gene that causes cells to divide more often (known as the Kras oncogene). Experimentally, the transformation of the duct-like cells into a cancerous form is modelled in mice that are engineered to express the Kras oncogene. These mice develop small pre-cancerous lesions in their pancreas that only arise from a small subset of the acinar cells, despite mutant Kras expression in all cells.
Krah et al. – who are based at the University of Utah, Vanderbilt Medical Center and University of Texas Southwestern Medical Center – sought to determine what normally protects against the Kras-mediated transformation in acinar cells; that is to say, the safety mechanisms that are bypassed during the formation of pre-cancerous lesions.
Since the first step of pancreatic cancer development is the ADM process that causes the acinar cells to become duct-like, Krah et al. hypothesized that preventing ADM from occurring may protect against Kras-mediated transformation. They reasoned that PTF1A, a protein that regulates the differentiation of acinar cells from precursor cells during pancreas development is similarly required to maintain the identity of the acinar cells in the adult pancreas. Thus, PTF1A could protect against the Kras-mediated transformation of the cells to a pre-cancerous cell type by preventing ADM. This hypothesis was based on previous studies of other molecules that regulate differentiation and guide the development of acinar cells, because these so-called “acinar differentiation factors” have been seen to protect against Kras-mediated transformation. Furthermore, inactivating these differentiation factors promotes the ADM process (Shi et al., 2009; von Figura et al., 2014).
To investigate the role of PTF1A, Krah et al. first examined when and where this protein was expressed throughout the pancreas. This revealed that PTF1A is not produced in the pre-cancerous lesions of either humans or mice. However, PTF1A was expressed in adjacent acinar cells that appeared normal, suggesting that PTF1A may protect against Kras-mediated transformation in these cells.
Next, Krah et al. used genetic mouse models to prevent acinar cells from producing PTF1A, and observed spontaneous ADM in these animals. Expressing the Kras oncogene in these mice caused precursor lesions to form more rapidly and over a wider area of the pancreas than those seen in mice whose acinar cells expressed both PTF1A and oncogenic Kras. Together, these data show that acinar cells must lose PTF1A before they can undergo ADM, and thus become susceptible to Kras-induced transformation.
Furthermore, by gene expression analysis, Krah et al. showed that PTF1A controls multiple genes and pathways associated with both the initiation of pancreatic cancer and their continuous need for oncogenic Kras expression.
Overall, Krah et al.'s data show that acinar cell differentiation, which is maintained by PTF1A, protects against the expression of oncogenic Kras and helps to prevent the cells from becoming cancerous. The next open question is whether forced expression of PTF1A in the pancreas protects against Kras-induced transformation; this has already been shown to be the case for a different acinar cell factor called Mist1 (Direnzo et al., 2012). Further open questions include what it is that causes the accelerated rate at which ADM progresses to precursor lesions in mice that express oncogenic Kras and lack PTF1A (compared with mice that do express PTF1A). Since PTF1A is normally lost during the process of ADM, the absence of this protein cannot explain the accelerated rate of cancer progression. It is possible that in mice that carry oncogenic Kras and still retain intact PTF1A, an equilibrium between acinar cells and ADM cells occurs, whereby ADM cells can ‘choose’ to re-express PTF1A and return to an acinar fate, or progress to form pre-cancerous lesions (Figure 1). This equilibrium would be lost in mice lacking PTF1A, where cells that have undergone ADM have no choice other than to progress towards becoming cancerous. Furthermore, the effect PTF1A re-expression has in pre-cancerous lesions as well as in advanced disease merits further exploration.
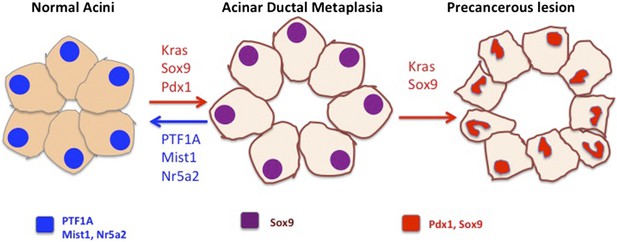
Preventing acinar cells differentiating to duct-like cells may help to prevent the development of pancreatic cancer.
In the figure, the cell nuclei are colour-coded to indicate which genes are expressed. Acinar cells in the pancreas (left) express the differentiation factors PTF1A, Mist1 and Nr5a2 (blue). Krah et al. show that loss of PTF1A causes acinar de-differentiation in a process known as acinar-to-ductal metaplasia (ADM; centre). In the presence of oncogenic Kras, ADM leads to the formation of precancerous lesions (right) and, in time, cancer. Other factors have also been shown to be important in regulating the balance between cell differentiation and cancer: Mist1 and Nr5a2, expressed in normal acinar cells, protect from transformation. Conversely, factors that control ductal fate, including Sox9 and Pdx1, can push acinar cells toward a ductal state and accelerate the rate at which cancerous cells develop (red arrows; Kopp et al., 2012; Miyatsuka et al., 2006).
In summary, this study provides new insight into the process that triggers the development of pancreatic cancer. In doing so, it opens many avenues of exploration that will provide more insight into the biology of this disease – an essential first step towards developing new therapies.
References
Article and author information
Author details
Publication history
- Version of Record published: August 14, 2015 (version 1)
Copyright
© 2015, Schofield and Pasca di Magliano
This article is distributed under the terms of the Creative Commons Attribution License, which permits unrestricted use and redistribution provided that the original author and source are credited.
Metrics
-
- 1,478
- views
-
- 148
- downloads
-
- 1
- citations
Views, downloads and citations are aggregated across all versions of this paper published by eLife.
Download links
Downloads (link to download the article as PDF)
Open citations (links to open the citations from this article in various online reference manager services)
Cite this article (links to download the citations from this article in formats compatible with various reference manager tools)
Further reading
-
- Developmental Biology
Vascular smooth muscle cells (VSMCs) envelop vertebrate brain arteries and play a crucial role in regulating cerebral blood flow and neurovascular coupling. The dedifferentiation of VSMCs is implicated in cerebrovascular disease and neurodegeneration. Despite its importance, the process of VSMC differentiation on brain arteries during development remains inadequately characterized. Understanding this process could aid in reprogramming and regenerating dedifferentiated VSMCs in cerebrovascular diseases. In this study, we investigated VSMC differentiation on zebrafish circle of Willis (CoW), comprising major arteries that supply blood to the vertebrate brain. We observed that arterial specification of CoW endothelial cells (ECs) occurs after their migration from cranial venous plexus to form CoW arteries. Subsequently, acta2+ VSMCs differentiate from pdgfrb+ mural cell progenitors after they were recruited to CoW arteries. The progression of VSMC differentiation exhibits a spatiotemporal pattern, advancing from anterior to posterior CoW arteries. Analysis of blood flow suggests that earlier VSMC differentiation in anterior CoW arteries correlates with higher red blood cell velocity and wall shear stress. Furthermore, pulsatile flow induces differentiation of human brain PDGFRB+ mural cells into VSMCs, and blood flow is required for VSMC differentiation on zebrafish CoW arteries. Consistently, flow-responsive transcription factor klf2a is activated in ECs of CoW arteries prior to VSMC differentiation, and klf2a knockdown delays VSMC differentiation on anterior CoW arteries. In summary, our findings highlight blood flow activation of endothelial klf2a as a mechanism regulating initial VSMC differentiation on vertebrate brain arteries.
-
- Developmental Biology
Precise developmental timing control is essential for organism formation and function, but its mechanisms are unclear. In C. elegans, the microRNA lin-4 critically regulates developmental timing by post-transcriptionally downregulating the larval-stage-fate controller LIN-14. However, the mechanisms triggering the activation of lin-4 expression toward the end of the first larval stage remain unknown. We demonstrate that the transmembrane transcription factor MYRF-1 is necessary for lin-4 activation. MYRF-1 is initially localized on the cell membrane, and its increased cleavage and nuclear accumulation coincide with lin-4 expression timing. MYRF-1 regulates lin-4 expression cell-autonomously and hyperactive MYRF-1 can prematurely drive lin-4 expression in embryos and young first-stage larvae. The tandem lin-4 promoter DNA recruits MYRF-1GFP to form visible loci in the nucleus, suggesting that MYRF-1 directly binds to the lin-4 promoter. Our findings identify a crucial link in understanding developmental timing regulation and establish MYRF-1 as a key regulator of lin-4 expression.