Post-translational Modifications: Reversing ADP-ribosylation
Cells rapidly react to stimuli in their environment by making modifications to proteins that change the way those proteins interact with other molecules (Mann and Jensen, 2003). Once a stimulus has stopped, these 'post-translational modifications' are usually reversed and the cell’s life goes back to normal. For example, when a cell suffers damage to its DNA, the addition of a molecule called ADP-ribose – a process that is known as ADP-ribosylation – to certain proteins sends a signal that leads to the damage being repaired; drugs that inhibit the addition of ADP-ribose are also used in cancer therapy (see Li and Yu, 2015 for a review).
It was discovered in the 1960s that specialized enzymes called PARPs can add one or more units of ADP-ribose (ADPr) to specific amino acids within proteins. Over the decades, it became clear that these enzymes are involved in a wide range of cellular processes, including DNA repair, transcription, chromatin regulation and cell death. The first target sites for ADP-ribosylation to be identified were mostly glutamates, aspartates and lysines, and the enzymes responsible for the removal of the ADPr units were also established (Figure 1)(Barkauskaite et al., 2013).
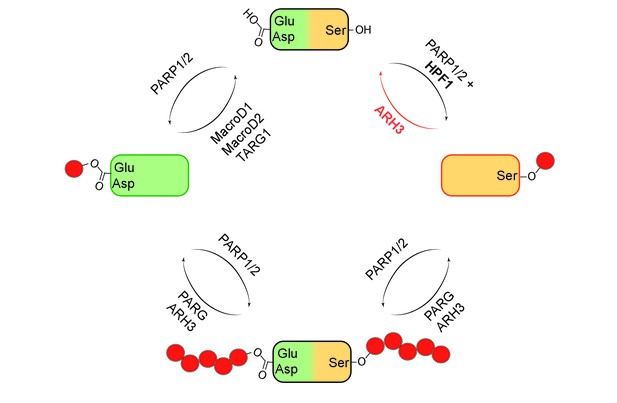
Mono- and poly(ADP-ribosyl)ation and their reversal.
When a protein (top) undergoes mono(ADP-ribosyl)ation the ADP-ribose (red circle) can be added to a glutamate (Glu) or aspartate (Asp; left) or a serine (Ser; right). It is also possible for multiple units of ADP-ribose to be added to a protein at a given target site in a process known as poly(ADP-ribosyl)ation (bottom). The enzymes PARP1 and PARP2 are involved in ADP-ribosylation of both Glu/Asp and Ser, with a protein called HPF1 acting as a cofactor in the mono(ADP-ribosyl)ation of Ser. The enzymes involved in the reversal of both mono- and poly(ADP-ribosyl)ation are shown. Fontana et al. have shown that ARH3 is exclusively responsible for reversing the mono(ADP-ribosyl)ation of Ser, and that it is also involved (with PARG) in reversing the poly(ADP-ribosyl)ation of Ser.
More recently, it was shown that serines can be target sites for ADP-ribosylation, and that many of the proteins that contain such target sites have important roles in DNA damage repair (Bilan et al., 2017; Bonfiglio et al., 2017; Leidecker et al., 2016; Gibbs-Seymour et al., 2016). However, nothing was known about the enzymes or mechanisms responsible for the removal of the ADPr units from the serines. Now, in eLife, Ivan Ahel of the University of Oxford, Ivan Matic of the Max Planck Institute for Biology of Ageing in Cologne and co-workers – including Pietro Fontana, Juan José Bonfiglio and Luca Palazzo as joint first authors, along with Edward Bartlett – provide new insight into these matters (Fontana et al., 2017).
Using biochemical approaches and a technique called mass spectrometry, Fontana et al. screened a number of proteins that are known to bind to ADPr to find out if they could remove ADPr units that had been added to serines. They discovered that an enzyme called ARH3 could remove ADPr from serine on histone proteins (Figure 1). Previous research has shown that ARH3 and PARG work in similar ways. Both enzymes are able to break the ribose bonds that hold chains of ADPr units together, but ARH3 hydrolyses the chains less efficiently than PARG and also has a different structure (Mueller-Dieckmann et al., 2006; Oka et al., 2006). Fontana et al. discovered that unlike PARG, ARH3 was able to cleave both single ADPr units and chains of ADPr on histones and other proteins.
Since mass spectrometry is a rather expensive and laborious technique, Fontana et al. also used ARH3 in combination with western blotting – a basic technique to detect specific proteins or protein modifications – to track ADP-ribosylation on serines. These experiments confirmed the findings obtained with mass spectrometry, and proved that histone proteins are primarily – if not exclusively – modified on serine. Future studies could build on these findings and use ARH3 as a tool to detect the ADP-ribosylation of serines in proteins.
Despite these new insights, many outstanding questions remain. For example, how does adding ADPr to serine affect the role of a protein? And what happens when two neighboring amino acids experience post-translational modifications? A widely studied post-translational modification that regulates gene expression involves the methylation or acetylation of two lysines (K9 and K27) in histone three (Saksouk et al., 2015). However, these lysines are followed by a serine, which could undergo its own post-translation modification (which could be phosphorylation or ADP-ribosylation). Would these modifications influence each other? Probably, yes. This complex interplay may have far reaching consequences in the regulation of gene expression, and may play an important role in many diseases that depend on ADP-ribosylation pathways.
References
-
The recognition and removal of cellular poly(ADP-ribose) signalsFEBS Journal 280:3491–3507.https://doi.org/10.1111/febs.12358
-
Serine ADP-ribosylation depends on HPF1Molecular Cell 65:932–940.https://doi.org/10.1016/j.molcel.2017.01.003
-
Serine is a new target residue for endogenous ADP-ribosylation on histonesNature Chemical Biology 12:998–1000.https://doi.org/10.1038/nchembio.2180
-
Proteomic analysis of post-translational modificationsNature Biotechnology 21:255–261.https://doi.org/10.1038/nbt0303-255
-
Identification and characterization of a mammalian 39-kDa poly(ADP-ribose) glycohydrolaseJournal of Biological Chemistry 281:705–713.https://doi.org/10.1074/jbc.M510290200
-
Constitutive heterochromatin formation and transcription in mammalsEpigenetics & Chromatin 8:3.https://doi.org/10.1186/1756-8935-8-3
Article and author information
Author details
Publication history
Copyright
© 2017, Moeller et al.
This article is distributed under the terms of the Creative Commons Attribution License, which permits unrestricted use and redistribution provided that the original author and source are credited.
Metrics
-
- 1,274
- views
-
- 145
- downloads
-
- 1
- citations
Views, downloads and citations are aggregated across all versions of this paper published by eLife.
Download links
Downloads (link to download the article as PDF)
Open citations (links to open the citations from this article in various online reference manager services)
Cite this article (links to download the citations from this article in formats compatible with various reference manager tools)
Further reading
-
- Biochemistry and Chemical Biology
The conformational ensemble and function of intrinsically disordered proteins (IDPs) are sensitive to their solution environment. The inherent malleability of disordered proteins, combined with the exposure of their residues, accounts for this sensitivity. One context in which IDPs play important roles that are concomitant with massive changes to the intracellular environment is during desiccation (extreme drying). The ability of organisms to survive desiccation has long been linked to the accumulation of high levels of cosolutes such as trehalose or sucrose as well as the enrichment of IDPs, such as late embryogenesis abundant (LEA) proteins or cytoplasmic abundant heat-soluble (CAHS) proteins. Despite knowing that IDPs play important roles and are co-enriched alongside endogenous, species-specific cosolutes during desiccation, little is known mechanistically about how IDP-cosolute interactions influence desiccation tolerance. Here, we test the notion that the protective function of desiccation-related IDPs is enhanced through conformational changes induced by endogenous cosolutes. We find that desiccation-related IDPs derived from four different organisms spanning two LEA protein families and the CAHS protein family synergize best with endogenous cosolutes during drying to promote desiccation protection. Yet the structural parameters of protective IDPs do not correlate with synergy for either CAHS or LEA proteins. We further demonstrate that for CAHS, but not LEA proteins, synergy is related to self-assembly and the formation of a gel. Our results suggest that functional synergy between IDPs and endogenous cosolutes is a convergent desiccation protection strategy seen among different IDP families and organisms, yet the mechanisms underlying this synergy differ between IDP families.
-
- Biochemistry and Chemical Biology
- Stem Cells and Regenerative Medicine
Human induced pluripotent stem cells (hiPSCs) have great potential to be used as alternatives to embryonic stem cells (hESCs) in regenerative medicine and disease modelling. In this study, we characterise the proteomes of multiple hiPSC and hESC lines derived from independent donors and find that while they express a near-identical set of proteins, they show consistent quantitative differences in the abundance of a subset of proteins. hiPSCs have increased total protein content, while maintaining a comparable cell cycle profile to hESCs, with increased abundance of cytoplasmic and mitochondrial proteins required to sustain high growth rates, including nutrient transporters and metabolic proteins. Prominent changes detected in proteins involved in mitochondrial metabolism correlated with enhanced mitochondrial potential, shown using high-resolution respirometry. hiPSCs also produced higher levels of secreted proteins, including growth factors and proteins involved in the inhibition of the immune system. The data indicate that reprogramming of fibroblasts to hiPSCs produces important differences in cytoplasmic and mitochondrial proteins compared to hESCs, with consequences affecting growth and metabolism. This study improves our understanding of the molecular differences between hiPSCs and hESCs, with implications for potential risks and benefits for their use in future disease modelling and therapeutic applications.