Noncoding RNA: Aging well with Norad
Aging is a complex process that affects most living organisms. Over a century of research has revealed that many different intrinsic and extrinsic factors contribute to our bodies growing old, but we are still a long way from a full understanding of all the mechanisms at play. Recent research has implicated molecules that do not code for proteins in this process, such as long noncoding RNAs. For instance, many of these so-called 'lncRNAs' are expressed differently in patients with age-related disorders, such as various forms of neurodegenerative disease and cancer (Rinn and Chang, 2012).
Now, in eLife, Joshua Mendell of the University of Texas Southwestern Medical Center (UTSW) and colleagues – including Florian Kopp as first author – report that losing the long noncoding RNA Norad accelerates aging in mice (Kopp et al., 2019). Previous studies have shown that Norad is highly conserved in mammals, and that it is abundant in most human cells (Lee et al., 2016; Tichon et al., 2016). The production of Norad increases in response to DNA damage and it helps preserve the genetic information as cells divide. Norad is mainly found in the cytoplasm, where it can bind two PUMILIO proteins (PUM1 and PUM2) that regulate a variety of targets in the cell, including some involved in cell growth and division (Figure 1). Yet, it was unknown what deleting Norad would entail at the level of an organism.
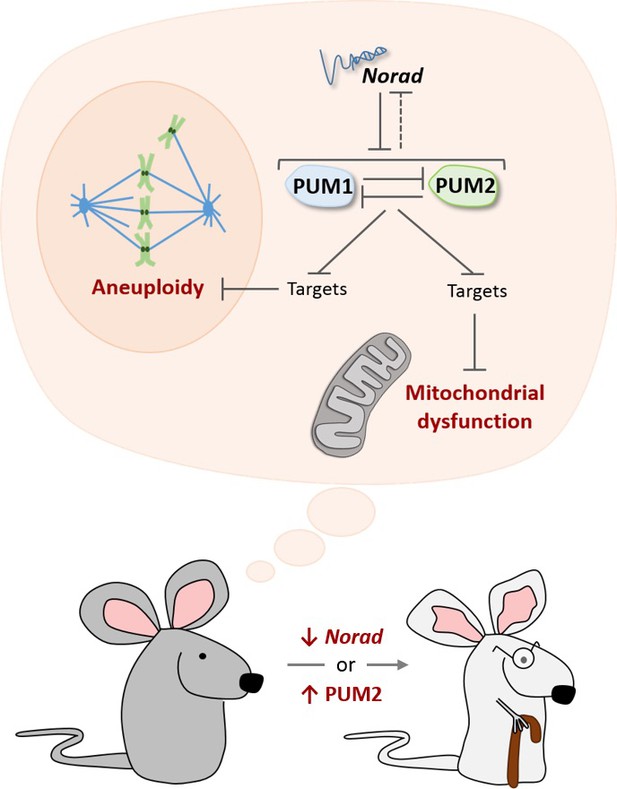
The Norad-PUMILIO axis.
The long noncoding RNA Norad (top) can bind to the PUMILIO proteins PUM1 (pale blue) and PUM2 (pale green) and repress their activity (Lee et al., 2016; Tichon et al., 2016). PUM1 and PUM2 also inhibit each other, and they may repress Norad (dotted inhibitory arrow; Goldstrohm et al., 2018). In mice that are genetically engineered to lack Norad or to overproduce PUM2, PUMILIO proteins become overactive and strongly inhibit molecules that prevent damage to mitochondria (bottom dark and light gray structure), as well as molecules that prevent cells from acquiring the wrong number of chromosomes (aneuploidy). As a result, these mice show accelerated aging (bottom; Kopp et al., 2019).
To investigate this question, Kopp et al. – who are based at UTSW, the Nationwide Children's Hospital and Ohio State University – engineered mice that lacked Norad. As the mutant animals grew, they displayed signs of premature aging: their fur thinned down and grayed faster; their spine showed the abnormal curvature associated with old age; their fat reserves dwindled more quickly; and they died earlier. Mice lacking Norad also exhibited several cellular hallmarks of aging (López-Otín et al., 2013). Many of their cells had the wrong number of chromosomes (as previously seen in Norad-deficient human cells), as well as mitochondria that were showing defects. This was associated with the repression of PUMILIO targets that keep mitochondria working properly. Strikingly, mice engineered to over-produce PUM2 had similar characteristics, clearly indicating that the hyperactivity of PUMILIO drives the symptoms associated with a lack of Norad (Figure 1).
On the flipside, a recent study in humans showed that halving the dose of PUM1 leads to developmental delay and seizures (Gennarino et al., 2018), and reducing the amount of PUM1, PUM2, or both results in smaller body size (Lin et al., 2019). These results suggest that mammals must maintain the activity of PUMILIO proteins within a narrow range in order to remain healthy. In fact, the levels of PUM2 proteins in bulk tissues are indistinguishable between control mice and animals that overexpress Pum2. Furthermore, in both Norad-deficient and Pum2-overexpressing mice, most PUMILIO targets are only affected very mildly, yet the animals exhibit striking phenotypes. Kopp et al. argue that repressing many PUMILIO targets at the same time, even weakly, may trigger the physiological damages observed in these rodents.
Still, it may also be possible that when the Norad-PUMILIO axis is disrupted, certain cells or tissues are more likely to stop working properly, which in turn creates a snowball effect for the whole organism. For instance, the expression of Norad is highest in the human brain, but it decreases with age in a region that acts as a reservoir to regenerate brain cells (Barry et al., 2015). In simpler organisms, such as the worm C. elegans, the nervous system acts on other tissues to coordinate pathways that keep proteins in their normal conformation, but these mechanisms become defective with age (van Oosten-Hawle and Morimoto, 2014). It remains unclear whether the nervous system performs such roles in mammals; yet, these results raise the possibility that interactions between Norad and PUMILIO are needed in specific tissues to ward off the effects of age.
Norad is PUMILIO’s preferred target, and has several binding sites that are recognized by these proteins. This suggests that this lncRNA can bind a large number of PUMILIO proteins at any given moment, but it is still unclear whether Norad tempers the activity of PUM1 and PUM2 simply by competing with other targets of PUMILIO, or if another mechanism is at play (Goldstrohm et al., 2018; Tichon et al., 2018).
Curiously, Norad is only found in mammals, whereas the PUMILIO proteins are found in all eukaryotes; it is therefore possible that RNAs with different sequences also buffer the activity of PUMILIO in other species. It remains to be seen how and why mammalian cells rely on Norad to tame the activity of these proteins, as opposed to other types of regulatory mechanisms. Ultimately, the work by Kopp et al. sets the ground for further enquiries into the role of noncoding RNAs and RNA-binding proteins in aging, which could potentially yield new therapeutic approaches for diseases brought by old age.
References
-
Post-transcriptional regulatory functions of mammalian Pumilio proteinsTrends in Genetics 34:972–990.https://doi.org/10.1016/j.tig.2018.09.006
-
Genome regulation by long noncoding RNAsAnnual Review of Biochemistry 81:145–166.https://doi.org/10.1146/annurev-biochem-051410-092902
Article and author information
Author details
Publication history
- Version of Record published: March 8, 2019 (version 1)
Copyright
© 2019, Marques and Ulitsky
This article is distributed under the terms of the Creative Commons Attribution License, which permits unrestricted use and redistribution provided that the original author and source are credited.
Metrics
-
- 2,471
- views
-
- 205
- downloads
-
- 6
- citations
Views, downloads and citations are aggregated across all versions of this paper published by eLife.
Download links
Downloads (link to download the article as PDF)
Open citations (links to open the citations from this article in various online reference manager services)
Cite this article (links to download the citations from this article in formats compatible with various reference manager tools)
Further reading
-
- Chromosomes and Gene Expression
- Developmental Biology
Developmental programming involves the accurate conversion of signalling levels and dynamics to transcriptional outputs. The transcriptional relay in the Notch pathway relies on nuclear complexes containing the co-activator Mastermind (Mam). By tracking these complexes in real time, we reveal that they promote the formation of a dynamic transcription hub in Notch ON nuclei which concentrates key factors including the Mediator CDK module. The composition of the hub is labile and persists after Notch withdrawal conferring a memory that enables rapid reformation. Surprisingly, only a third of Notch ON hubs progress to a state with nascent transcription, which correlates with polymerase II and core Mediator recruitment. This probability is increased by a second signal. The discovery that target-gene transcription is probabilistic has far-reaching implications because it implies that stochastic differences in Notch pathway output can arise downstream of receptor activation.
-
- Chromosomes and Gene Expression
Eukaryotic chromatin is organized into functional domains, that are characterized by distinct proteomic compositions and specific nuclear positions. In contrast to cellular organelles surrounded by lipid membranes, the composition of distinct chromatin domains is rather ill described and highly dynamic. To gain molecular insight into these domains and explore their composition, we developed an antibody-based proximity-biotinylation method targeting the RNA and proteins constituents. The method that we termed Antibody-Mediated-Proximity-Labelling-coupled to Mass Spectrometry (AMPL-MS) does not require the expression of fusion proteins and therefore constitutes a versatile and very sensitive method to characterize the composition of chromatin domains based on specific signature proteins or histone modifications. To demonstrate the utility of our approach we used AMPL-MS to characterize the molecular features of the chromocenter as well as the chromosome territory containing the hyperactive X-chromosome in Drosophila. This analysis identified a number of known RNA binding proteins in proximity of the hyperactive X and the centromere, supporting the accuracy of our method. In addition, it enabled us to characterize the role of RNA in the formation of these nuclear bodies. Furthermore, our method identified a new set of RNA molecules associated with the Drosophila centromere. Characterization of these novel molecules suggested the formation of R-loops in centromeres, which we validated using a novel probe for R-loops in Drosophila. Taken together, AMPL-MS improves the selectivity and specificity of proximity ligation allowing for novel discoveries of weak protein-RNA interactions in biologically diverse domains.