Spermatogenesis: A hotspot for new genes
New genes can be produced in a number of different ways. Existing genes can be duplicated, while frameshift mutations may change the way a cell reads a sequence, which could lead to new proteins. 'De novo' genes can also be created from previously non-coding sequences. It was thought until recently that the emergence of these genes was extremely rare, but the advent of modern genomics and transcriptomics has revealed that this is not the case (Schlötterer, 2015; Jacob, 1977). In multicellular organisms, only a small proportion of the genome codes for proteins, yet a large fraction of the rest of the genome is actively transcribed and translated (Clark et al., 2011; Ruiz-Orera et al., 2018). These non-coding sequences provide the raw material for natural selection to act upon and create new genes: considering that most of the genome is non-coding in multicellular organisms, the potential for de novo genes to emerge is enormous.
De novo genes tend to be expressed in the testis and involved in male reproductive processes (Levine et al., 2006; Begun et al., 2007; Zhao et al., 2014). However, many of these genes are not fixed within a species, which means they are susceptible to disappear. In addition, a large number of genes associated with male reproduction are rapidly evolving (Swanson et al., 2001). These observations are surprising given that sperm formation is a complex, highly conserved process. It is possible that de novo genes arise more often in the testis because future sperm cells have a permissive DNA state which would allow transcription to proceed less specifically than in other tissues, exposing non-coding sequences to selection (Kaessmann, 2010). However, this hypothesis is notoriously difficult to test because the testis is a highly heterogeneous tissue: for example, germ cells pass through many different stages before they mature into sperm.
Single-cell RNA sequencing is a powerful technology that circumvents the challenges associated with tissue heterogeneity by revealing the expression profile of individual cells. It can be harnessed to study mixed cell populations in tumors, or to track transcriptional dynamics during complex developmental processes such as sperm formation. This mechanism, also known as spermatogenesis, requires a pool of germ stem cells to undergo a series of mitotic and meiotic divisions to finally produce mature sperm (Figure 1). Now, in eLife, Li Zhao and colleagues at the Rockefeller University – including Evan Witt as first author, Sigi Benjamin and Nicolas Svetec – report having leveraged single-cell RNA sequencing to investigate how de novo genes are expressed in fly testis during the different stages of spermatogenesis (Witt et al., 2019).
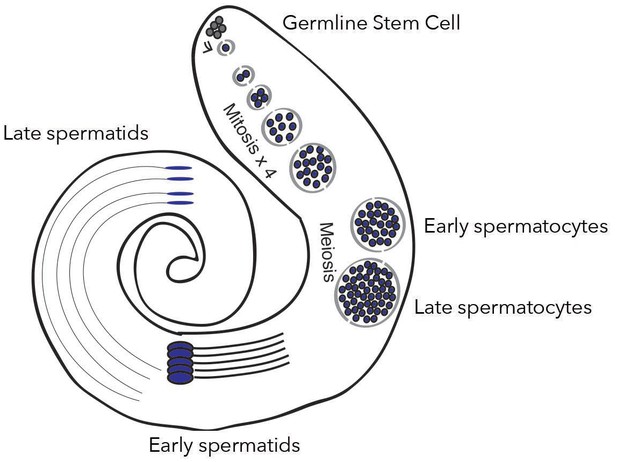
New genes are expressed differently depending on spermatogenesis stages.
In the testis of fruit flies, the creation of mature sperm, or spermatogenesis, starts with a germline stem cell going through several rounds of mitosis to form early spermatocytes. After meiosis, these cells become late spermatocytes, which then develop into early and late spermatids. Witt et al. show that fixed de novo genes, which emerge from non-coding sequences, are expressed during mid-spermatogenesis, in particular in early spermatocytes. In contrast, other types of new genes, for example which come from gene duplication, are expressed at different stages.
Image credit: Witt et al., 2019; adapted from Figure 1A (CC BY 4.0).
First, the Rockefeller team was able to categorize the cells as germline stem cells, somatic cells, or cells going through specific stages of maturation by analyzing the expression of well-known spermatogenesis marker genes. In addition, the researchers used overall transcriptional changes to reconstruct an inferred ‘pseudotime’, a roadmap of the different stages that cells go through as they develop into sperm. Together, these approaches allowed Witt et al. to thoroughly document the gene expression profiles of specific cell types during spermatogenesis.
In particular, they found that fixed de novo genes (that is, de novo genes that are unlikely to disappear from a species) were expressed differently depending on developmental stage. For example, they were more often switched on in meiotic germ cells, a pattern reminiscent of canonical genes expressed only in testes. However, unfixed de novo genes were less expressed in germline stem cells, and enriched in early sperm cells (which have gone through meiosis). These results indicate that de novo genes could be expressed during meiosis, and that the expression pattern of a de novo gene may impact its probability to reach fixation.
Next, Witt et al. compared the expression profiles of de novo and recent duplicate genes. The expression pattern of almost half of the derived duplicate genes was biased towards early and late spermatogenesis, a pattern never observed for parental genes. On the contrary, the expression of the majority of de novo genes was biased towards mid-spermatogenesis. These observations suggest that de novo and young duplicate genes are regulated differently, and that the mode of emergence of a new gene constrains how it is expressed.
Finally, Witt et al. developed a method to infer the presence of mutations from single-cell RNA sequencing data. Combined with the cell type and pseudotime information, this allowed them to estimate the mutational load – the amount of potentially deleterious mutations – over spermatogenesis. They found that the mutational load tends to decrease over pseudotime, suggesting that DNA damage was repaired or that carrier cells were eliminated. While this finding requires further validation, it opens the door to many questions related to DNA repair dynamics, selection pressure within the testis and even male fertility.
As evolution largely relies on the mutation rate in the germline, the work by Witt et al. elegantly highlights how single-cell sequencing technologies can start to address long-standing questions in evolutionary biology. One of the upcoming challenges is now to integrate these highly multidimensional data with the complex molecular pathways involved in reproduction and development.
References
-
The reality of pervasive transcriptionPLOS Biology 9:e1000625.https://doi.org/10.1371/journal.pbio.1000625
-
Origins, evolution, and phenotypic impact of new genesGenome Research 20:1313–1326.https://doi.org/10.1101/gr.101386.109
-
Translation of neutrally evolving peptides provides a basis for de novo gene evolutionNature Ecology & Evolution 2:890–896.https://doi.org/10.1038/s41559-018-0506-6
-
Genes from scratch--the evolutionary fate of de novo genesTrends in Genetics 31:215–219.https://doi.org/10.1016/j.tig.2015.02.007
Article and author information
Author details
Publication history
Copyright
© 2019, Dion-Côté
This article is distributed under the terms of the Creative Commons Attribution License, which permits unrestricted use and redistribution provided that the original author and source are credited.
Metrics
-
- 2,848
- views
-
- 268
- downloads
-
- 6
- citations
Views, downloads and citations are aggregated across all versions of this paper published by eLife.
Download links
Downloads (link to download the article as PDF)
Open citations (links to open the citations from this article in various online reference manager services)
Cite this article (links to download the citations from this article in formats compatible with various reference manager tools)
Further reading
-
- Evolutionary Biology
Lineages of rod-shaped bacteria such as Escherichia coli exhibit a temporal decline in elongation rate in a manner comparable to cellular or biological aging. The effect results from the production of asymmetrical daughters, one with a lower elongation rate, by the division of a mother cell. The slower daughter compared to the faster daughter, denoted respectively as the old and new daughters, has more aggregates of damaged proteins and fewer expressed gene products. We have examined further the degree of asymmetry by measuring the density of ribosomes between old and new daughters and between their poles. We found that ribosomes were denser in the new daughter and also in the new pole of the daughters. These ribosome patterns match the ones we previously found for expressed gene products. This outcome suggests that the asymmetry is not likely to result from properties unique to the gene expressed in our previous study, but rather from a more fundamental upstream process affecting the distribution of ribosomal abundance. Because damage aggregates and ribosomes are both more abundant at the poles of E. coli cells, we suggest that competition for space between the two could explain the reduced ribosomal density in old daughters. Using published values for aggregate sizes and the relationship between ribosomal number and elongation rates, we show that the aggregate volumes could in principle displace quantitatively the amount of ribosomes needed to reduce the elongation rate of the old daughters.
-
- Evolutionary Biology
- Genetics and Genomics
Evolutionary arms races can arise at the contact surfaces between host and viral proteins, producing dynamic spaces in which genetic variants are continually pursued. However, the sampling of genetic variation must be balanced with the need to maintain protein function. A striking case is given by protein kinase R (PKR), a member of the mammalian innate immune system. PKR detects viral replication within the host cell and halts protein synthesis to prevent viral replication by phosphorylating eIF2α, a component of the translation initiation machinery. PKR is targeted by many viral antagonists, including poxvirus pseudosubstrate antagonists that mimic the natural substrate, eIF2α, and inhibit PKR activity. Remarkably, PKR has several rapidly evolving residues at this interface, suggesting it is engaging in an evolutionary arms race, despite the surface’s critical role in phosphorylating eIF2α. To systematically explore the evolutionary opportunities available at this dynamic interface, we generated and characterized a library of 426 SNP-accessible nonsynonymous variants of human PKR for their ability to escape inhibition by the model pseudosubstrate inhibitor K3, encoded by the vaccinia virus gene K3L. We identified key sites in the PKR kinase domain that harbor K3-resistant variants, as well as critical sites where variation leads to loss of function. We find K3-resistant variants are readily available throughout the interface and are enriched at sites under positive selection. Moreover, variants beneficial against K3 were also beneficial against an enhanced variant of K3, indicating resilience to viral adaptation. Overall, we find that the eIF2α-binding surface of PKR is highly malleable, potentiating its evolutionary ability to combat viral inhibition.