Brain Development: Why the young sleep longer
From absorbing new languages to mastering musical instruments, young children are wired to learn in ways that adults are not (Johnson and Newport, 1989). This ability coincides with periods of intense brain plasticity during which neurons can easily remodel their connections (Hubel and Wiesel, 1970). Many children are also scandalously good sleepers, typically getting several more hours of sleep per night than their parents (Jenni and Carskadon, 2007). As sleep deprivation has negative effects on learning and memory, learning like a child likely requires sleeping like one (Diekelmann and Born, 2010). Yet, how the ability to sleep for longer is synchronized with windows of high brain plasticity is not fully understood.
Sleep is deeply conserved through evolution, and examining how it develops in ‘simple organisms’ should provide fundamental insights relevant to humans. For instance, like human teenagers, one-day old Drosophila melanogaster flies sleep twice as much as mature adults, and disrupting the sleep of young flies has lasting effects on learning and behavior (Seugnet et al., 2011; Kayser et al., 2014). Now, in eLife, Matthew Kayser from the University of Pennsylvania and co-workers – including Leela Chakravarti Dilley as first author – report new regulatory mechanisms that promote sleep in young flies (Chakravarti Dilley et al., 2020).
The team started by searching for genes which, when knocked down, would reduce the difference in sleep duration between younger and older adult flies. A gene called pdm3 fit the bill by reducing sleep in juveniles. This gene codes for a transcription factor that belongs to a family known to regulate normal brain development. Chakravarti Dilley et al. further determined that pdm3 helps to establish correct sleep patterns for one-day-old flies during the pupal stage, when the relatively simple brain of a larva develops into the complex brain of the adult insect – a period of radical change that puts human puberty to shame.
Previous work on pdm3 mutants revealed aberrations in the way dopaminergic neurons reach and connect with neurons in the central complex, a region of the brain that is known to regulate sleep. There, the dopaminergic neurons encourage wakefulness by inhibiting cells called dFSB neurons, which promote sleep (Pimentel et al., 2016). In flies, the density of connections between dopaminergic and dFSB neurons normally increases over the first few days of adult life. Chakravarti Dilley et al. therefore explored whether pdm3 might regulate how dopaminergic neurons innervate the central complex. This revealed that when pdm3 was knocked down, one-day-old flies already showed levels of innervation that rivaled those seen in mature adults (Figure 1).
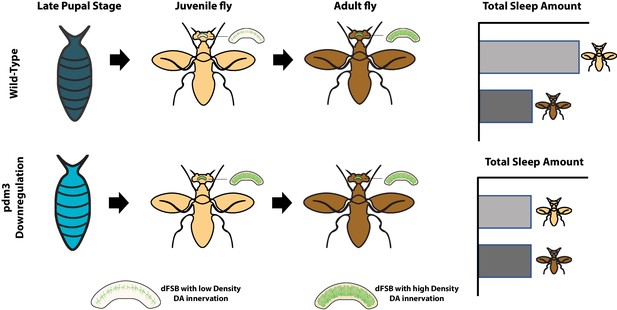
How the transcription factor pdm3 preserves high levels of sleep in young flies.
Expression of pdm3 during the pupal stage (top row) delays the innervation of the dFSB neurons (which promote sleep) by dopaminergic neurons (green) that encourage wakefulness. The progressive innervation of these neurons as the fly ages results in adult flies (dark) spending less time asleep than young flies (pale). Knock down of pdm3 (bottom row) results in premature innervation, leading to young flies spending much less time asleep. dFSB: dorsal fan-shaped body; DA: dopaminergic.
Given that pdm3 encodes a transcription factor, the team then searched for genes that regulate sleep and whose expression was altered by pdm3 being knocked down. These experiments suggested that pdm3 suppresses the expression of msp300, a gene from a family involved in synapse maturation. And indeed, knocking down both pdm3 and msp300 resulted in flies that developed normally in terms of sleep patterns and dopaminergic innervation of the central complex.
Perturbing neural development, especially during windows of high plasticity, can have a long-lasting impact on the ability for the brain to work properly (Marín, 2016). A lack of sleep could lead to such perturbations, as evidenced by the fact that disrupting sleep in early childhood or adolescence has long-term effects on behavior (Taveras et al., 2017; Roberts et al., 2009). Understanding how sleep is synchronized with periods of intense development may help to develop better therapeutic interventions that lessen long-term brain damage.
References
-
The memory function of sleepNature Reviews Neuroscience 11:114–126.https://doi.org/10.1038/nrn2762
-
The period of susceptibility to the physiological effects of unilateral eye closure in kittensThe Journal of Physiology 206:419–436.https://doi.org/10.1113/jphysiol.1970.sp009022
-
Sleep behavior and sleep regulation from infancy through adolescence: normative aspectsSleep Medicine Clinics 2:321–329.https://doi.org/10.1016/j.jsmc.2007.05.001
-
Sleepless in adolescence: prospective data on sleep deprivation, health and functioningJournal of Adolescence 32:1045–1057.https://doi.org/10.1016/j.adolescence.2009.03.007
Article and author information
Author details
Publication history
- Version of Record published: April 27, 2020 (version 1)
Copyright
© 2020, Chowdhury and Shafer
This article is distributed under the terms of the Creative Commons Attribution License, which permits unrestricted use and redistribution provided that the original author and source are credited.
Metrics
-
- 1,917
- views
-
- 157
- downloads
-
- 2
- citations
Views, downloads and citations are aggregated across all versions of this paper published by eLife.
Download links
Downloads (link to download the article as PDF)
Open citations (links to open the citations from this article in various online reference manager services)
Cite this article (links to download the citations from this article in formats compatible with various reference manager tools)
Further reading
-
- Chromosomes and Gene Expression
- Genetics and Genomics
Members of the diverse heterochromatin protein 1 (HP1) family play crucial roles in heterochromatin formation and maintenance. Despite the similar affinities of their chromodomains for di- and tri-methylated histone H3 lysine 9 (H3K9me2/3), different HP1 proteins exhibit distinct chromatin-binding patterns, likely due to interactions with various specificity factors. Previously, we showed that the chromatin-binding pattern of the HP1 protein Rhino, a crucial factor of the Drosophila PIWI-interacting RNA (piRNA) pathway, is largely defined by a DNA sequence-specific C2H2 zinc finger protein named Kipferl (Baumgartner et al., 2022). Here, we elucidate the molecular basis of the interaction between Rhino and its guidance factor Kipferl. Through phylogenetic analyses, structure prediction, and in vivo genetics, we identify a single amino acid change within Rhino’s chromodomain, G31D, that does not affect H3K9me2/3 binding but disrupts the interaction between Rhino and Kipferl. Flies carrying the rhinoG31D mutation phenocopy kipferl mutant flies, with Rhino redistributing from piRNA clusters to satellite repeats, causing pronounced changes in the ovarian piRNA profile of rhinoG31D flies. Thus, Rhino’s chromodomain functions as a dual-specificity module, facilitating interactions with both a histone mark and a DNA-binding protein.
-
- Genetics and Genomics
- Neuroscience
Cognitive decline is a significant health concern in our aging society. Here, we used the model organism C. elegans to investigate the impact of the IIS/FOXO pathway on age-related cognitive decline. The daf-2 Insulin/IGF-1 receptor mutant exhibits a significant extension of learning and memory span with age compared to wild-type worms, an effect that is dependent on the DAF-16 transcription factor. To identify possible mechanisms by which aging daf-2 mutants maintain learning and memory with age while wild-type worms lose neuronal function, we carried out neuron-specific transcriptomic analysis in aged animals. We observed downregulation of neuronal genes and upregulation of transcriptional regulation genes in aging wild-type neurons. By contrast, IIS/FOXO pathway mutants exhibit distinct neuronal transcriptomic alterations in response to cognitive aging, including upregulation of stress response genes and downregulation of specific insulin signaling genes. We tested the roles of significantly transcriptionally-changed genes in regulating cognitive functions, identifying novel regulators of learning and memory. In addition to other mechanistic insights, a comparison of the aged vs young daf-2 neuronal transcriptome revealed that a new set of potentially neuroprotective genes is upregulated; instead of simply mimicking a young state, daf-2 may enhance neuronal resilience to accumulation of harm and take a more active approach to combat aging. These findings suggest a potential mechanism for regulating cognitive function with age and offer insights into novel therapeutic targets for age-related cognitive decline.