Mutations: A larger target leads to faster evolution
Evolution is a two-step process: first, variation is generated, for example through random mutations; and then events such as natural selection determine whether specific traits become more or less common in a population. Some traits evolve faster than others, and different explanations for this emphasize the relative importance of one or the other of the two steps. For example, a fast-evolving trait could be under sustained and intense selection pressure, so that when a favorable new variant arises, it quickly spreads throughout the population. Alternatively, mutations might affect certain traits more than others, leading to more variation in these traits. Since variation is the fuel of evolution, this could make the affected traits change faster.
At the molecular level, two mechanisms can bias which traits are more likely to be affected by mutations. On one hand, the genes that affect a particular trait could be located in genomic areas with high mutation rates, known as ‘mutational hotspots’ (Fondon and Garner, 2004; Xie et al., 2019); on the other, a phenotype could depend on a large number genes, increasing the ‘mutational target size’ (Boyle et al., 2017).
Caenorhabditis elegans and C. briggsae worms have two sexes – males, which are very rare, and hermaphrodites. In hermaphrodites, the cell divisions and fate decisions that occur during development are nearly identical for individuals within a species. However, a cell called P3.p, which is involved in the development of the vulva, sometimes divides and sometimes does not, even among worms with the same genome (Sulston and Horvitz, 1977). A decade ago Marie-Anne Félix and colleagues reported that the frequency of this binary decision evolves more quickly than the cell fate decisions made by other early vulval cells, and that this fast evolution was probably due more to mutational biases – either hotspots or large target size – than selection effects (Braendle et al., 2010). Now, in eLife, Fabrice Besnard, Joao Picao-Osorio, Clément Dubois and Félix – who are based at laboratories in Lyon and Paris – report that this mutational bias is caused by a large mutational target size (Besnard et al., 2020).
To show this, Besnard et al. used mutation accumulation lines of either C. elegans or the closely related C. briggsae. These lines started from the offspring of a single individual and were bred in parallel. All of the lines were inbred: in each line a single selfing hermaphrodite was the parent of the next generation. This meant that if the parent had a new mutation, there would be a good chance that its offspring would inherit it. In this environment, the effects of natural selection were minimized because the only requirement for a line to endure was that a single hermaphrodite from that line survived and reproduced. After many generations, each line accumulated its own constellation of new mutations. Since the lines all started out genetically identical and selection was minimal, any differences in traits between the lines were be due to the different mutations.
The mutation accumulation lines used in these experiments were evolved for 250 generations (Baer et al., 2005), after which Besnard et al. chose to look at five lines in which the P3.p cell fate changed the most. By combining genomic sequencing and gene modification through CRISPR technology, they identified one gene in each line that was responsible for the change and showed that: (i) none of the genes are in a mutational hotspot in the genome; and (ii) only one of these genes was known to have a role specifically in vulval development. This suggests that the mutational target for P3.p cell fate is much broader than previously thought (Figure 1).
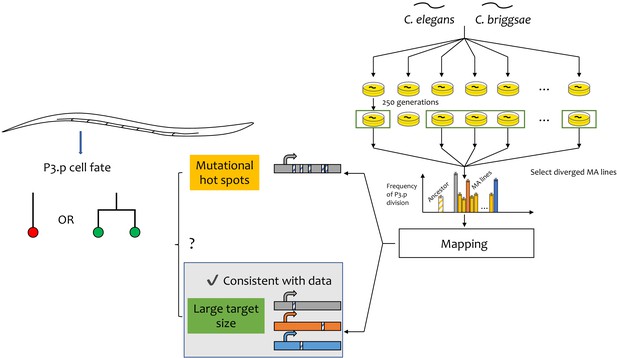
Fast evolution of a cell-fate decision in C. elegans and C. briggsae.
The vulva in Caenorhabditis worms develops from six precursor cells. In some individuals, the most anterior of these cells (called P3.p) divides during development; in other worms it does not (left). The chance of it dividing differs between species. Cell division in the other five vulval precursor cells (VPCs; left) does not vary. This faster evolution of P3.p may be due due to mutational hotspots (if the genes that regulate this decision have a relatively high mutation rate) or to a large target size (if there are relatively more genes that affect this decision). Besnard et al. used a mutation accumulation (MA) approach in two species – C. elegans and C. briggsae – to distinguish between the two explanations (right). They examined the MA lines in which the decision frequency differed the most from the ancestor (shown by the vertical grey, orange and blue bars in the graph) and identified the genes responsible (shown by the horizontal grey, orange and blue bars at the bottom of the figure). They found that none of these genes appear to be in a mutational hotspot and that they have a range of different biological roles. In this case, fast evolution is due to a large mutational target size.
This broad mutational target is consistent with many genome-wide association studies and genetic mapping studies (Manolio et al., 2009; Shi et al., 2016). These experiments suggest that trait evolution tends not to be caused by one or two mutations with very large effects: rather, tens (or even hundreds) of genes carrying mutations with small effects seem to be responsible. These previous studies also suggest, rather unexpectedly, that a large fraction of the genes that underlie trait variation often have very little or no previously known functional relationships to the traits, and this is also the case in the work of Besnard et al. Therefore, there is a discrepancy between genetic pathways that have been described in the last century as being responsible for specific traits and the genes whose variation apparently fuels evolution.
The use of Caenorhabditis species in this work reinforces the power of this model system in research on developmental and evolutionary biology. Because so much is known about worm cell fates, Besnard et al. were able to go beyond identifying genes and pose testable hypotheses for why this particular cell was more variable than other vulval precursors. For example, they point out that the P3.p cell is at the far end of a gradient of cell fate-inducing molecules that are secreted from the tail end of the animal. The Félix lab previously showed that P3.p cell division is much more sensitive to variations in the dose of these molecules than cells closer to the source of the gradient (Pénigault and Félix, 2011). This suggests that the decision by P3.p to divide or not operates close to a concentration threshold for these molecules. This in turn means that mutations with small effects on the shape of the gradient, or on the responsiveness of P3.p to the molecules, could have large effects on the fate of the P3.p cell.
Developmental processes are responsible for generating the effects of many mutations. Therefore, to fully understand how mutations influence evolution, we must first understand the developmental context in which they occur.
References
-
Evolution of a system sensitive to stochastic noise: p3.p cell fate in CaenorhabditisDevelopmental Biology 357:419–427.https://doi.org/10.1016/j.ydbio.2011.05.675
-
Contrasting the genetic architecture of 30 complex traits from summary association dataThe American Journal of Human Genetics 99:139–153.https://doi.org/10.1016/j.ajhg.2016.05.013
-
Post-embryonic cell lineages of the nematode, Caenorhabditis elegansDevelopmental Biology 56:110–156.https://doi.org/10.1016/0012-1606(77)90158-0
Article and author information
Author details
Publication history
Copyright
© 2020, Yang and Rifkin
This article is distributed under the terms of the Creative Commons Attribution License, which permits unrestricted use and redistribution provided that the original author and source are credited.
Metrics
-
- 2,553
- views
-
- 120
- downloads
-
- 0
- citations
Views, downloads and citations are aggregated across all versions of this paper published by eLife.
Download links
Downloads (link to download the article as PDF)
Open citations (links to open the citations from this article in various online reference manager services)
Cite this article (links to download the citations from this article in formats compatible with various reference manager tools)
Further reading
-
- Ecology
- Evolutionary Biology
Eurasia has undergone substantial tectonic, geological, and climatic changes throughout the Cenozoic, primarily associated with tectonic plate collisions and a global cooling trend. The evolution of present-day biodiversity unfolded in this dynamic environment, characterised by intricate interactions of abiotic factors. However, comprehensive, large-scale reconstructions illustrating the extent of these influences are lacking. We reconstructed the evolutionary history of the freshwater fish family Nemacheilidae across Eurasia and spanning most of the Cenozoic on the base of 471 specimens representing 279 species and 37 genera plus outgroup samples. Molecular phylogeny using six genes uncovered six major clades within the family, along with numerous unresolved taxonomic issues. Dating of cladogenetic events and ancestral range estimation traced the origin of Nemacheilidae to Indochina around 48 mya. Subsequently, one branch of Nemacheilidae colonised eastern, central, and northern Asia, as well as Europe, while another branch expanded into the Burmese region, the Indian subcontinent, the Near East, and northeast Africa. These expansions were facilitated by tectonic connections, favourable climatic conditions, and orogenic processes. Conversely, aridification emerged as the primary cause of extinction events. Our study marks the first comprehensive reconstruction of the evolution of Eurasian freshwater biodiversity on a continental scale and across deep geological time.
-
- Evolutionary Biology
Gene duplication drives evolution by providing raw material for proteins with novel functions. An influential hypothesis by Ohno (1970) posits that gene duplication helps genes tolerate new mutations and thus facilitates the evolution of new phenotypes. Competing hypotheses argue that deleterious mutations will usually inactivate gene duplicates too rapidly for Ohno’s hypothesis to work. We experimentally tested Ohno’s hypothesis by evolving one or exactly two copies of a gene encoding a fluorescent protein in Escherichia coli through several rounds of mutation and selection. We analyzed the genotypic and phenotypic evolutionary dynamics of the evolving populations through high-throughput DNA sequencing, biochemical assays, and engineering of selected variants. In support of Ohno’s hypothesis, populations carrying two gene copies displayed higher mutational robustness than those carrying a single gene copy. Consequently, the double-copy populations experienced relaxed purifying selection, evolved higher phenotypic and genetic diversity, carried more mutations and accumulated combinations of key beneficial mutations earlier. However, their phenotypic evolution was not accelerated, possibly because one gene copy rapidly became inactivated by deleterious mutations. Our work provides an experimental platform to test models of evolution by gene duplication, and it supports alternatives to Ohno’s hypothesis that point to the importance of gene dosage.