HIV-1 Infection: You can keep your coat on
Viruses are pathogens that rely on hosts to produce multiple copies of themselves. Retroviruses, such as HIV-1, have a unique way of integrating their genes into the DNA of a host cell. Since their genetic information is stored as RNA, a retrovirus must first undergo a process called reverse transcription. This process, which is catalyzed by an enzyme called reverse transcriptase, is followed by a second step called integration (catalyzed by integrase), which results in the viral DNA being inserted into the genome of the host cell. Although the mechanical functions of these two enzymes are well understood, relatively little is known about the transition between reverse transcription and integration during HIV-1 infection.
Much of the research into these processes has centered on the capsid protein, which self-associates to form a honeycomb-like container that surrounds the genetic material inside the retrovirus (Bedwell and Engelman, 2021). Once the retrovirus has entered a cell, the capsid container protects the RNA and the two enzymes from the antiviral molecular machinery in the cell cytoplasm, allowing reverse transcription to proceed without interruption (Yamashita and Engelman, 2017). At the same time, various cellular proteins bind to the outside of the capsid in order to facilitate it being trafficked to the nucleus, where the DNA of the host cell resides.
Once reverse transcription has been completed, the capsid is degraded to release the viral DNA from its protective container. Until recently, it was assumed that this process – which is called uncoating – might be a prerequisite for the viral DNA to enter the nucleus because it was thought that the HIV-1 capsid was too large to pass through the nuclear pore complex (which is the doorway through which biomolecules pass to enter the nucleus; Yamashita and Engelman, 2017; Arhel et al., 2007; Francis and Melikyan, 2018). However, recent studies have found evidence for capsid lattices or intact capsid-like structures inside the nucleus (Burdick et al., 2020; Dharan et al., 2020; Selyutina et al., 2020), with one study even suggesting that the intact capsid is able to pass through the nuclear pore complex (Zila et al., 2021; Figure 1). Now, in eLife, Hans-Georg Kräusslich and colleagues at the University Hospital Heidelberg and the German Center for Infection Research – including Thorsten Müller as first author – report new details on the timing, localization and mechanism of HIV-1 uncoating (Müller et al., 2021).
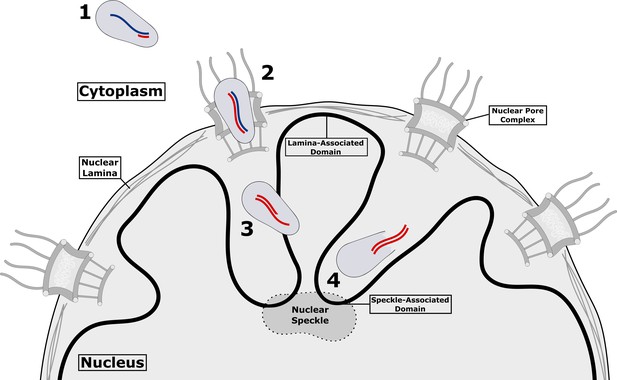
HIV-1 inside a host cell.
After HIV-1 has reached the cytoplasm of the host cell (1), reverse transcription begins within the virus capsid, which is still intact: viral RNA in shown in blue, and the newly synthesized viral DNA is shown in red. Reverse transcription continues as the capsid passes through the nuclear pore complex (2) and into the nucleus (3). Once the process of reverse transcription has been completed, the forces exerted by the double-stranded DNA (double red lines) compromise the structural integrity of the capsid, creating a gap through which the DNA can escape (4) and go on to be integrated in the DNA of the host cell (not shown). The breakdown of the capsid and release of the DNA is referred to as uncoating. Integration occurs in chromatin (solid black line) near nuclear speckles (speckle-associated domains) and away from nuclear lamina (lamina-associated domains). It is currently unclear if there are similar preferred sites for uncoating.
Müller et al. used different fluorescent labels to identify distinct events during HIV-1 replication. The ANCHOR labeling system – which employs a fluorescently labeled bacterial protein to bind a specific DNA sequence engineered into the HIV-1 genome – enabled them to distinguish between the initial reverse-transcription products made comparatively early during infection, and the more complete, double-stranded DNA that is accessible only after uncoating has happened. Müller et al. also tagged the incoming virus with a fluorescently labeled integrase protein in order to study the kinetics of uncoating. These experiments revealed that complete viral DNA was mostly tagged after nuclear import, suggesting that the capsid remained intact as it entered the nucleus, and that uncoating took place thereafter. Moreover, uncoating began in the nucleus as early as eight hours after infection with HIV-1 (Figure 1).
These observations suggest little – if any – loss of the capsid protein prior to nuclear import. Consistent with this interpretation, high-resolution imaging techniques revealed intact capsid-like structures at sites close to ANCHOR-labeled viral DNA. Tomographic reconstructions of these structures provided a unique snapshot of the HIV-1 uncoating process, showing what appeared to be holes in capsid-like structures that did not contain viral DNA. This quite striking finding is consistent with a previous model of uncoating in which reverse transcription leads to the generation of mechanical forces that compromise the structural integrity of the capsid (Figure 1; Rankovic et al., 2017). In many ways, the capsid is like an egg from which the integration-competent viral DNA is hatched!
The work of Müller et al. fills in some of the gaps in our understanding of the early stages of HIV-1 replication. We now have a clearer picture of how and when the final integration-competent complex can be released from the capsid shell. An important next step will be to unravel the link between uncoating and integration.
In recent years, it has become clear that integration tends to take place at chromatin regions that are close to structures called nuclear speckles and away from the nuclear lamina (Figure 1; Bedwell and Engelman, 2021). It is possible, perhaps even likely, that uncoating also occurs at or near these same chromatin regions. Alternatively, a post-uncoating trafficking event might help guide the integration complex to these regions. A theory that can link reverse transcription, uncoating and integration will clarify the paths taken during HIV-1 infection which, in turn, could inform the development of new antiviral strategies.
References
-
Factors that mold the nuclear landscape of HIV-1 integrationNucleic Acids Research 49:621–635.https://doi.org/10.1093/nar/gkaa1207
-
Reverse transcription mechanically initiates HIV-1 capsid disassemblyJournal of Virology 91:e00289.https://doi.org/10.1128/JVI.00289-17
-
Capsid-dependent host factors in HIV-1 infectionTrends in Microbiology 25:741–755.https://doi.org/10.1016/j.tim.2017.04.004
Article and author information
Author details
Publication history
Copyright
© 2021, Bedwell and Engelman
This article is distributed under the terms of the Creative Commons Attribution License, which permits unrestricted use and redistribution provided that the original author and source are credited.
Metrics
-
- 853
- views
-
- 70
- downloads
-
- 0
- citations
Views, downloads and citations are aggregated across all versions of this paper published by eLife.
Download links
Downloads (link to download the article as PDF)
Open citations (links to open the citations from this article in various online reference manager services)
Cite this article (links to download the citations from this article in formats compatible with various reference manager tools)
Further reading
-
- Cell Biology
Endometriosis is a debilitating disease affecting 190 million women worldwide and the greatest single contributor to infertility. The most broadly accepted etiology is that uterine endometrial cells retrogradely enter the peritoneum during menses, implant and form invasive lesions in a process analogous to cancer metastasis. However, over 90% of women suffer retrograde menstruation, but only 10% develop endometriosis, and debate continues as to whether the underlying defect is endometrial or peritoneal. Processes implicated in invasion include: enhanced motility; adhesion to, and formation of gap junctions with, the target tissue. Endometrial stromal (ESCs) from 22 endometriosis patients at different disease stages show much greater invasiveness across mesothelial (or endothelial) monolayers than ESCs from 22 control subjects, which is further enhanced by the presence of EECs. This is due to enhanced responsiveness of endometriosis ESCs to the mesothelium, which induces migration and gap junction coupling. ESC-PMC gap junction coupling is shown to be required for invasion, while coupling between PMCs enhances mesothelial barrier breakdown.
-
- Cell Biology
How the fate (folding versus degradation) of glycoproteins is determined in the endoplasmic reticulum (ER) is an intriguing question. Monoglucosylated glycoproteins are recognized by lectin chaperones to facilitate their folding, whereas glycoproteins exposing well-trimmed mannoses are subjected to glycoprotein ER-associated degradation (gpERAD); we have elucidated how mannoses are sequentially trimmed by EDEM family members (George et al., 2020; 2021 eLife). Although reglucosylation by UGGT was previously reported to have no effect on substrate degradation, here we directly tested this notion using cells with genetically disrupted UGGT1/2. Strikingly, the results showed that UGGT1 delayed the degradation of misfolded substrates and unstable glycoproteins including ATF6α. An experiment with a point mutant of UGGT1 indicated that the glucosylation activity of UGGT1 was required for the inhibition of early glycoprotein degradation. These and overexpression-based competition experiments suggested that the fate of glycoproteins is determined by a tug-of-war between structure formation by UGGT1 and degradation by EDEMs. We further demonstrated the physiological importance of UGGT1, since ATF6α cannot function properly without UGGT1. Thus, our work strongly suggests that UGGT1 is a central factor in ER protein quality control via the regulation of both glycoprotein folding and degradation.