Photosynthesis: Oxygen overload
Bioreactors of algae are commonly used to generate biomass, a renewable source of energy. To do this, scientists exploit the algae’s ability to efficiently convert carbon dioxide into organic compounds, such as sugar, using photosynthesis. This process is known as carbon fixation and requires high levels of inorganic carbon in order to be successful. If the primary enzyme involved in this reaction – called Rubisco (short for ribulose-1,5-bisphosphate carboxylase/oxygenase) – does not receive enough carbon dioxide, it mistakenly fixes oxygen instead. This results in unfavourable by-products that inhibit photosynthesis and require lots of energy to recycle (Peterhansel et al., 2010).
To overcome this problem, algae evolved a carbon dioxide concentrating mechanism (CCM) that allows the rate of photosynthesis to remain high despite low levels of inorganic carbon (Badger and Price, 1992; Raven et al., 2017). Much of what is known about the CCM has come from studying a single cell alga known as Chlamydomonas (Mackinder et al., 2017). These studies revealed two key components that allow the CCM to increase the concentration of carbon dioxide around Rubisco enzymes: transporter proteins that pump carbon dioxide into the chloroplast, and structures called pyrenoids which sequester Rubisco enzymes. Pyrenoids are condensed proteins which are often surrounded by a starch sheath that promotes the selective entry of carbon dioxide and shields Rubisco from oxygen. However, the mechanisms and signals underlying the formation of these pyrenoids have largely remained elusive.
Past and current research has mostly focused on pyrenoids that appear when CCMs are induced by low levels of inorganic carbon (Wang et al., 2015). Now, in eLife, David M Kramer (Michigan State University) and colleagues – including Peter Neofotis (also from Michigan State) as first author – report that pyrenoids may also be induced by high levels of oxygen, in the absence of other CCM components (Neofotis et al., 2021).
The team – which included researchers from Michigan State and ExxonMobil – found that a species of alga called Chlamydomonas reinhardtii contains pyrenoids when there are extremely high levels of oxygen in the air (also known as hyperoxia) despite carbon dioxide levels also being high (Figure 1). The structure of these oxygen-induced pyrenoids is strikingly similar to those formed in response to low levels of inorganic carbon. This led Neofotis et al. to hypothesize that the signal that induces pyrenoid formation may be produced when photosynthesis takes place either under hyperoxia conditions or when there are low levels of carbon dioxide.
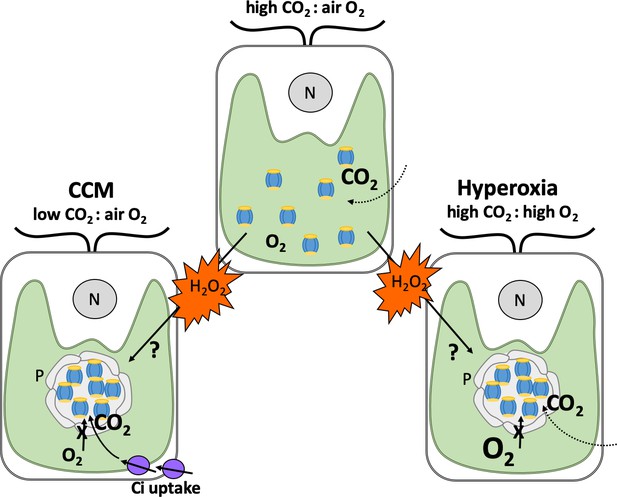
How hydrogen peroxide stimulates the formation of pyrenoids under different conditions.
Depicted are typical Chlamydomonas cells with a single cup-shaped chloroplast (green) and a nucleus (grey circle, N). When carbon dioxide (CO2) levels in the air surrounding the alga are higher than oxygen (O2; top), Rubisco enzymes (blue and yellow) are dispersed in the chloroplast and efficiently fix carbon dioxide. When carbon dioxide levels are lower than oxygen (left), Rubisco fixes oxygen instead. This leads to harmful metabolites that need to be recycled, resulting in the generation of the by-product hydrogen peroxide (H2O2; orange). To counteract this, Chlamydomonas employ a carbon dioxide concentrating mechanism (CCM) which sequesters Rubisco enzymes in to pyrenoids that preclude the entry of oxygen (grey circular structure), and produces proteins that transport carbon dioxide into the chloroplast (Ci; purple). When oxygen levels are high (also known as hyperoxia; right), this leads to oxygen fixation and production of hydrogen peroxide as well. Neofotis et al. found that Chlamydomonas also contain pyrenoids under these conditions, even when there are high amounts of carbon dioxide and the full CCM is suppressed. This led them to propose that hydrogen peroxide triggers the formation of pyrenoids via an unknown mechanism when oxygen levels are high and when there are insufficient amounts of carbon dioxide.
Image credit: Britta Foerster (CC BY 4.0)
The signalling molecule hydrogen peroxide was considered to be the strongest candidate for the role as it is a by-product of oxygen fixation, a scenario that can occur when oxygen levels are high or when there are insufficient amounts of carbon dioxide (Figure 1). Further experiments showed that hydrogen peroxide levels and the formation of pyrenoids are highly correlated, providing strong evidence for this hypothesis.
To address the role pyrenoids play under high oxygen stress, Neofotis et al. studied and compared Chlamydomonas strains which have varying tolerance to hyperoxia. The experiments showed that the pyrenoids found in these genetic variants have different structures and sometimes fail to form a sheath. Algae that could endure high levels of oxygen and formed fully starch-sheathed pyrenoids had significant growth advantages over those that had more fragmented and poorly developed sheaths. This is consistent with the theory that pyrenoids also help to enhance photosynthesis under hyperoxia conditions, in addition to their role in the CCM.
Many questions remain to be answered to fully understand how hydrogen peroxide signalling leads to the formation of pyrenoids, regardless of whether low levels of inorganic carbon or hyperoxia are the eliciting cue. It is possible that other signals may affect the formation of pyrenoids, perhaps in response to other environmental stresses. Furthermore, how pyrenoids assemble and the specific roles of each of their different parts still remain to be explored. Nevertheless, the work of Neofotis et al. sets the scene for new, exciting avenues of research that may lead to the development of more efficient algae that can be used in bioreactors and other biotechnological applications.
References
-
The CO2 concentrating mechanism in cyanobacteria and microalgaePhysiologia Plantarum 84:606–615.https://doi.org/10.1111/j.1399-3054.1992.tb04711.x
-
The possible evolution and future of CO2-concentrating mechanismsJournal of Experimental Botany 68:3701–3716.https://doi.org/10.1093/jxb/erx110
Article and author information
Author details
Publication history
Copyright
© 2021, Foerster
This article is distributed under the terms of the Creative Commons Attribution License, which permits unrestricted use and redistribution provided that the original author and source are credited.
Metrics
-
- 990
- views
-
- 101
- downloads
-
- 0
- citations
Views, downloads and citations are aggregated across all versions of this paper published by eLife.
Download links
Downloads (link to download the article as PDF)
Open citations (links to open the citations from this article in various online reference manager services)
Cite this article (links to download the citations from this article in formats compatible with various reference manager tools)
Further reading
-
- Biochemistry and Chemical Biology
- Structural Biology and Molecular Biophysics
Riboswitches represent a class of non-coding RNA that possess the unique ability to specifically bind ligands and, in response, regulate gene expression. A recent report unveiled a type of riboswitch, known as the guanidine-IV riboswitch, which responds to guanidine levels to regulate downstream genetic transcription. However, the precise molecular mechanism through which the riboswitch senses its target ligand and undergoes conformational changes remain elusive. This gap in understanding has impeded the potential applications of this riboswitch. To bridge this knowledge gap, our study investigated the conformational dynamics of the guanidine-IV riboswitch RNA upon ligand binding. We employed single-molecule fluorescence resonance energy transfer (smFRET) to dissect the behaviors of the aptamer, terminator, and full-length riboswitch. Our findings indicated that the aptamer portion exhibited higher sensitivity to guanidine compared to the terminator and full-length constructs. Additionally, we utilized Position-specific Labelling of RNA (PLOR) combined with smFRET to observe, at the single-nucleotide and single-molecule level, the structural transitions experienced by the guanidine-IV riboswitch during transcription. Notably, we discovered that the influence of guanidine on the riboswitch RNA’s conformations was significantly reduced after the transcription of 88 nucleotides. Furthermore, we proposed a folding model for the guanidine-IV riboswitch in the absence and presence of guanidine, thereby providing insights into its ligand-response mechanism.
-
- Biochemistry and Chemical Biology
The biosynthesis of cyclic 3′,5′-adenosine monophosphate (cAMP) by mammalian membrane-bound adenylyl cyclases (mACs) is predominantly regulated by G-protein-coupled receptors (GPCRs). Up to now the two hexahelical transmembrane domains of mACs were considered to fix the enzyme to membranes. Here, we show that the transmembrane domains serve in addition as signal receptors and transmitters of lipid signals that control Gsα-stimulated mAC activities. We identify aliphatic fatty acids and anandamide as receptor ligands of mAC isoforms 1–7 and 9. The ligands enhance (mAC isoforms 2, 3, 7, and 9) or attenuate (isoforms 1, 4, 5, and 6) Gsα-stimulated mAC activities in vitro and in vivo. Substitution of the stimulatory membrane receptor of mAC3 by the inhibitory receptor of mAC5 results in a ligand inhibited mAC5–mAC3 chimera. Thus, we discovered a new class of membrane receptors in which two signaling modalities are at a crossing, direct tonic lipid and indirect phasic GPCR–Gsα signaling regulating the biosynthesis of cAMP.