Defense Proteins: Exposing the invader
The immune system is a large network of organs, cells and molecules that work together to protect the body from disease-causing pathogens. It consists of an innate immune response (which activates macrophages and dendritic cells) and an adaptive immune response (which activates T cells and B cells).
Most cells in the body also have an intrinsic response against viruses, in which they express proteins that can detect the invading virus and block its replication. From the initial entry into a cell, through to the budding and release of new virus particles, intrinsic anti-viral factors can detect a broad range of viral molecules, including the RNA or DNA of the virus. In response, cells produce signaling proteins, such as interferons, to stimulate anti-viral defenses in neighboring cells and to target viral infections at various stages of their lifecycle.
For example, the restriction factor myxovirus resistance protein B (MxB) is activated by interferons, and has been shown to inhibit the replication of a broad range of viruses, including HIV and herpesviruses (Liu et al., 2013; Matreyek et al., 2014; Crameri et al., 2018; Schilling et al., 2018). However, its mechanism of action is poorly understood (Wilbourne and Zhang, 2021; Xie et al., 2021). Now, in eLife, Beate Sodeik and colleagues – including Manutea Serrero from Hanover Medical School as first author – report new insights into the interactions between MxB and herpesviruses (Serrero et al., 2022).
All herpesviruses consist of a capsid protein that surrounds their DNA, and a layer called the tegument that links the capsid with the membrane envelope engulfing the virus particle (Figure 1A; Dai and Zhou, 2018; Huet et al., 2016). Upon infection, the viral envelope fuses with the membrane of the host cell and releases the capsid and most of the tegument layer into the cell. These are then transported to the nucleus, where they can hijack the replication machinery of the host cell (Figure 1B).
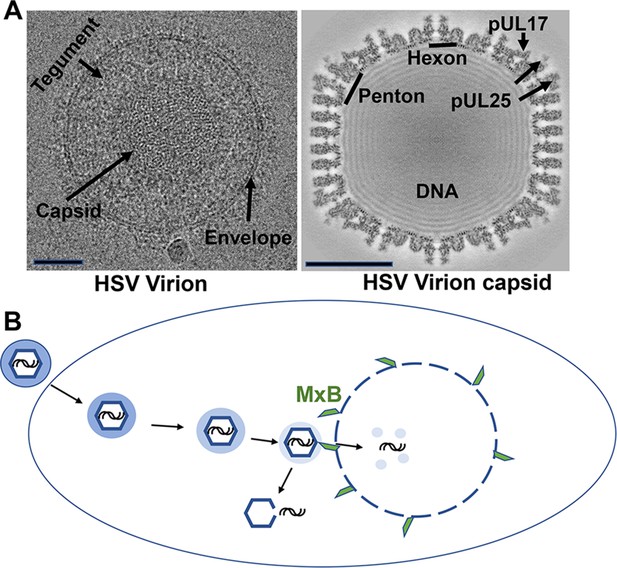
The structure and mechanisms of a herpes simplex virus.
(A) Cryo-electron microscopy images of a herpes simplex virus (left) and a section through a three-dimensional reconstruction of the viral capsid (right). Herpesviruses consist of a protective capsid containing the viral DNA, and a tegument layer connecting the capsid and the membrane envelope surrounding the virus. Two subunits, hexon and penton, are located at the icosahedral vertices of the capsid, where three proteins (pUL17, pUL25, pUL36 – the latter is not visible on the map) form a complex that anchors the tegument layer to the capsid. Scale bars shown on lower left of each image represent 50 nanometers. (B) Schematic representation showing entry of the herpes virus into cells, following the fusion of the viral envelope with the host cell membrane. Once inside the cell, the capsid (blue hexagon) and its associated tegument proteins (shaded blue ring) traffic to the nucleus along microtubules, and dock at the pores of the cell nucleus to release the viral genome (black lines). The interferon-induced protein MxB (green) can only attach to the capsid when the tegument is absent (light blue shading) and divert the viral genome into the host’s cytoplasm by seemingly punching holes into the vertices of the capsid. The exposed viral genome may alert defense proteins to activate an anti-viral response.
To find out how anti-viral proteins inhibit the replication of herpes simplex virus, Serrero et al. used cell-free methods to reconstitute the complexes typically formed by these proteins and viral particles. To do so, they prepared protein extracts from ‘naïve’ macrophages and macrophages that had been exposed to an interferon, as these cells are known to mount a robust interferon response. These were then mixed with either capsids from extracellular viral particles containing varying amounts of tegument proteins, or tegument-free capsids from the nuclei of infected cells. The resulting complexes were characterized using quantitative mass spectrometry. This revealed MxB as one of the molecules interacting with the capsid, with a particular affinity for capsids lacking the tegument layer.
To test if MxB could also affect the stability of the capsid, the team (who are based at various institutes in Germany, the United States and the United Kingdom) bound the virus capsids onto electron microscopy grids and incubated them with cellular fluids extracted from naïve macrophages or macrophages that had been exposed to interferons. Electron microscopy revealed that capsids treated with the interferon-stimulated cell extracts were significantly more damaged and impaired compared to the capsids exposed to untreated cell extracts.
Moreover, further experiments using host cells from genetically modified lung tissue lacking MxB showed that extracts from these cells did not damage the capsid, highlighting its importance in capsid breakdown. In particular, MxB may attach to connector proteins (pUL17, pUL25, pUL36) that normally link the tegument and the capsid (Figure 1A). Furthermore, Serrero et al. found that when MxB was added to extracts from naïve cells that had not been activated by interferons, this caused the capsids to breakdown.
Perhaps the most intriguing result is that MxB works most efficiently on capsids lacking the tegument layer. In fact, MxB was unable to bind to capsids and destroy them when tegument proteins remained associated with the capsid. This suggests that tegument proteins shield the capsids from immune factors such as MxB, potentially by blocking their interaction with the connector proteins pUL17, pUL25 and pUL36.
The study of Serrero et al. further suggests that there is a fine balance within cells where loss of tegument proteins determines when capsids can be targeted by anti-viral factors, such as MxB. The binding of MxB to the tegument-free capsid and its partial destruction may in turn affect how new virus particles target the host cell’s nuclei. This could potentially disrupt the assembly of future capsids formed during viral replication, as MxB might engage with virions as they mature in the nucleus. In addition, the release of viral genomes from the damaged capsids may enhance the activation of defense proteins sensing the foreign particle, thereby amplifying the intrinsic immune responses of the host cell.
Since these experiments were done using cell-free assays, it remains to be seen whether MxB induces the breakdown of viral capsids in infected cells. A better understanding of how the tegument can shield the capsids from MxB could pave the way for new treatments against herpesviruses and other viral invaders.
References
-
MxB is an interferon-induced restriction factor of human herpesvirusesNature Communications 9:1980.https://doi.org/10.1038/s41467-018-04379-2
-
Extensive subunit contacts underpin herpesvirus capsid stability and interior-to-exterior allosteryNature Structural & Molecular Biology 23:531–539.https://doi.org/10.1038/nsmb.3212
-
The interferon-inducible MxB protein inhibits HIV-1 infectionCell Host & Microbe 14:398–410.https://doi.org/10.1016/j.chom.2013.08.015
-
Human MxB protein is a pan-herpesvirus restriction factorJournal of Virology 92:e01056-18.https://doi.org/10.1128/JVI.01056-18
Article and author information
Author details
Publication history
Copyright
© 2022, Bayer and Homa
This article is distributed under the terms of the Creative Commons Attribution License, which permits unrestricted use and redistribution provided that the original author and source are credited.
Metrics
-
- 1,195
- views
-
- 85
- downloads
-
- 1
- citations
Views, downloads and citations are aggregated across all versions of this paper published by eLife.
Download links
Downloads (link to download the article as PDF)
Open citations (links to open the citations from this article in various online reference manager services)
Cite this article (links to download the citations from this article in formats compatible with various reference manager tools)
Further reading
-
- Immunology and Inflammation
Type 1 diabetes mellitus (T1DM), known as insulin-dependent diabetes mellitus, is characterized by persistent hyperglycemia resulting from damage to the pancreatic β cells and an absolute deficiency of insulin, leading to multi-organ involvement and a poor prognosis. The progression of T1DM is significantly influenced by oxidative stress and apoptosis. The natural compound eugenol (EUG) possesses anti-inflammatory, anti-oxidant, and anti-apoptotic properties. However, the potential effects of EUG on T1DM had not been investigated. In this study, we established the streptozotocin (STZ)-induced T1DM mouse model in vivo and STZ-induced pancreatic β cell MIN6 cell model in vitro to investigate the protective effects of EUG on T1DM, and tried to elucidate its potential mechanism. Our findings demonstrated that the intervention of EUG could effectively induce the activation of nuclear factor E2-related factor 2 (NRF2), leading to an up-regulation in the expressions of downstream proteins NQO1 and HMOX1, which are regulated by NRF2. Moreover, this intervention exhibited a significant amelioration in pancreatic β cell damage associated with T1DM, accompanied by an elevation in insulin secretion and a reduction in the expression levels of apoptosis and oxidative stress-related markers. Furthermore, ML385, an NRF2 inhibitor, reversed these effects of EUG. The present study suggested that EUG exerted protective effects on pancreatic β cells in T1DM by attenuating apoptosis and oxidative stress through the activation of the NRF2 signaling pathway. Consequently, EUG holds great promise as a potential therapeutic candidate for T1DM.
-
- Computational and Systems Biology
- Immunology and Inflammation
Transcription factor partners can cooperatively bind to DNA composite elements to augment gene transcription. Here, we report a novel protein-DNA binding screening pipeline, termed Spacing Preference Identification of Composite Elements (SPICE), that can systematically predict protein binding partners and DNA motif spacing preferences. Using SPICE, we successfully identified known composite elements, such as AP1-IRF composite elements (AICEs) and STAT5 tetramers, and also uncovered several novel binding partners, including JUN-IKZF1 composite elements. One such novel interaction was identified at CNS9, an upstream conserved noncoding region in the human IL10 gene, which harbors a non-canonical IKZF1 binding site. We confirmed the cooperative binding of JUN and IKZF1 and showed that the activity of an IL10-luciferase reporter construct in primary B and T cells depended on both this site and the AP1 binding site within this composite element. Overall, our findings reveal an unappreciated global association of IKZF1 and AP1 and establish SPICE as a valuable new pipeline for predicting novel transcription binding complexes.