A chronic signaling TGFb zebrafish reporter identifies immune response in melanoma
Abstract
Developmental signaling pathways associated with growth factors such as TGFb are commonly dysregulated in melanoma. Here we identified a human TGFb enhancer specifically activated in melanoma cells treated with TGFB1 ligand. We generated stable transgenic zebrafish with this TGFb Induced Enhancer driving green fluorescent protein (TIE:EGFP). TIE:EGFP was not expressed in normal melanocytes or early melanomas but was expressed in spatially distinct regions of advanced melanomas. Single-cell RNA-sequencing revealed that TIE:EGFP+ melanoma cells down-regulated interferon response while up-regulating a novel set of chronic TGFb target genes. ChIP-sequencing demonstrated that AP-1 factor binding is required for activation of chronic TGFb response. Overexpression of SATB2, a chromatin remodeler associated with tumor spreading, showed activation of TGFb signaling in early melanomas. Confocal imaging and flow cytometric analysis showed that macrophages localize to TIE:EGFP+ regions and preferentially phagocytose TIE:EGFP+ melanoma cells compared to TIE:EGFP- melanoma cells. This work identifies a TGFb induced immune response and demonstrates the need for the development of chronic TGFb biomarkers to predict patient response to TGFb inhibitors.
Editor's evaluation
This is an important study that discovered a TGFb-inducible enhancer region in a human melanoma cell line that functions across vertebrates and was used to generate a zebrafish melanoma TGFb reporter model. The data is solid and provides interesting insights into TGFb signaling that is only activated in advanced melanoma. The study also shows TGFb reporter-positive melanoma cells are preferentially phagocytosed by macrophages, making this paper of interest to biologists studying melanoma and cancer immunotherapy.
https://doi.org/10.7554/eLife.83527.sa0Introduction
Melanoma, arising from pigment producing melanocytes, is the deadliest form of skin cancer, with an estimated 100,640 new cases and 8,290 deaths in the United States in 2024 alone (Siegel et al., 2024). The most common mutation in melanoma is BRAFV600E, which accounts for approximately 50% of melanoma cases and results in activation of the MAPK pathway promoting cell growth and survival (Akbani et al., 2015; Lo and Fisher, 2014). In addition, developmental signaling pathways are commonly dysregulated. Melanoma cells have increased expression and secretion of TGFb ligands compared to normal melanocytes, and TGFb ligand expression correlates with melanoma progression (Van Belle et al., 1996; Albino et al., 1991; Rodeck et al., 1991; Rodeck et al., 1994; Krasagakis et al., 1998; Javelaud et al., 2008; Perrot et al., 2013; Rak et al., 1996; Cerami et al., 2012; Gao et al., 2013; Lauden et al., 2014; Schmid et al., 1995). TGFb ligand binding to receptors on the cell surface results in phosphorylation and activation of SMAD2 and SMAD3 transcription factors. SMAD2 and SMAD3 translocate to the nucleus with SMAD4 to modulate gene expression (Batlle and Massagué, 2019). In normal melanocytes and early melanoma, TGFb acts as a tumor suppressor. However, in advanced melanoma TGFb is pro-tumorigenic as it induces growth, invasion, and metastasis (Perrot et al., 2013). As current targeted MAPK and immune checkpoint inhibitors often result in resistance, there is a need to study additional pathways perturbed in melanoma, such as TGFb.
Most cells in the tumor microenvironment can respond to and initiate TGFb signaling, although this often occurs in a heterogenous manner (Derynck et al., 2021). Generally, TGFb has an immunosuppressive effect in advanced tumors, resulting in inactivation of cytotoxic CD8+ T cells, expansion of immune suppressive regulatory T cells, inhibition of dendritic cell antigen presentation, and conversion of macrophages to an anti-inflammatory and pro-angiogenic M2-like state (Batlle and Massagué, 2019; Derynck et al., 2021; Naganuma et al., 1996; Ahmadzadeh and Rosenberg, 2005; Donkor et al., 2011; Fridlender et al., 2009; Mantovani et al., 2002; Standiford et al., 2011; Kobie et al., 2003). TGFb can act as a chemoattractant for macrophages and monocytes to areas of inflammation. Recruitment of monocytes by TGFb results in differentiation into macrophages that attach to the extracellular matrix (ECM) or promote blood vessel leakiness allowing for tumor cell extravasation (Arwert et al., 2018; Kelly et al., 2018; Kim et al., 2021; Allen et al., 1990; Wahl et al., 1993). In colorectal and urothelial cancers, TGFb in the tumor microenvironment was found to mediate immune evasion such that TGFb inhibition rendered these tumors susceptible to anti-PD-1/PD-L1 immune checkpoint inhibitors (Tauriello et al., 2018; Mariathasan et al., 2018). Due to its immunosuppressive effect, several TGFb inhibitors are in clinical trials in combination with immune checkpoint inhibitors (Lan et al., 2018; Paz-Ares et al., 2020; Strauss et al., 2018; Martin et al., 2020; Welsh et al., 2021).
Here, we visualized TGFb response across zebrafish melanoma tumorigenesis. A human melanoma enhancer, induced upon TGFB1 signaling was identified, and stable transgenic zebrafish with this enhancer driving EGFP were generated (TIE:EGFP). This TGFb inducible enhancer reporter is expressed in spatially distinct regions in advanced zebrafish melanomas and is characterized by up-regulation of a series of novel chronic TGFb target genes involved in the extracellular matrix. Single-cell RNA-seq and confocal microscopy revealed that TIE:EGFP+ melanoma cells down-regulate interferon response and are preferentially phagocytosed by macrophages. Overexpression of the chromatin remodeler SATB2, which is associated with tumor spreading, shows early activation of TGFb signaling in these melanomas, suggesting that specific melanoma genotypes may benefit from TGFb inhibition. Overall, this work demonstrates the need for biomarker development to predict response to TGFb inhibitors in advanced or aggressive melanoma subtypes.
Results
A TGFb enhancer reporter is inducible and specific in zebrafish
To visualize dynamic TGFb response across melanoma development, we designed a TGFb Inducible Enhancer (TIE) reporter using human melanoma cell (A375) ChIP-seq data following a 2-hr TGFB1 treatment. RNA-sequencing indicated that following a 2-hr treatment of A375 cells with 10 ng/mL human recombinant TGFB1, 223 genes were significantly up-regulated (q<0.05) including typical TGFb target genes SMAD7, JUNB, and PMEPA1, while 94 genes were down-regulated (Figure 1—figure supplement 2A, left). Hallmark gene set enrichment analysis (GSEA) of genes ranked by log2 fold-change (log2fc) confirmed that TGFb was the top up-regulated pathway following 2-hr treatment (q=0) (Figure 1—figure supplement 2A, right). This indicates that a 2-hr treatment with human recombinant TGFB1 ligand is sufficient to activate the TGFb pathway.
To identify a TGFB1 inducible enhancer region, we selected a region of chromatin that was exclusively open upon stimulation, based on H3K27ac ChIP-seq, with unique SMAD2/3 binding following treatment (Figure 1A). The enhancer region under the SMAD2/3 peak was cloned upstream of a beta-globin minimal promoter and EGFP in a Tol2 vector backbone. TIE:EGFP was tested for inducibility in the presence of ubiquitously expressed constitutively active SMAD2 and SMAD3 (ubi:caSMAD2/3) by electroporation of adult zebrafish skin, with ubiquitous BFP (ubi:BFP) as a control for electroporation efficiency. TIE:EGFP reporter activity was significantly increased in the presence of ubi:caSMAD2 and ubi:caSMAD3, confirming that the reporter is inducible (Figure 1—figure supplement 1A).
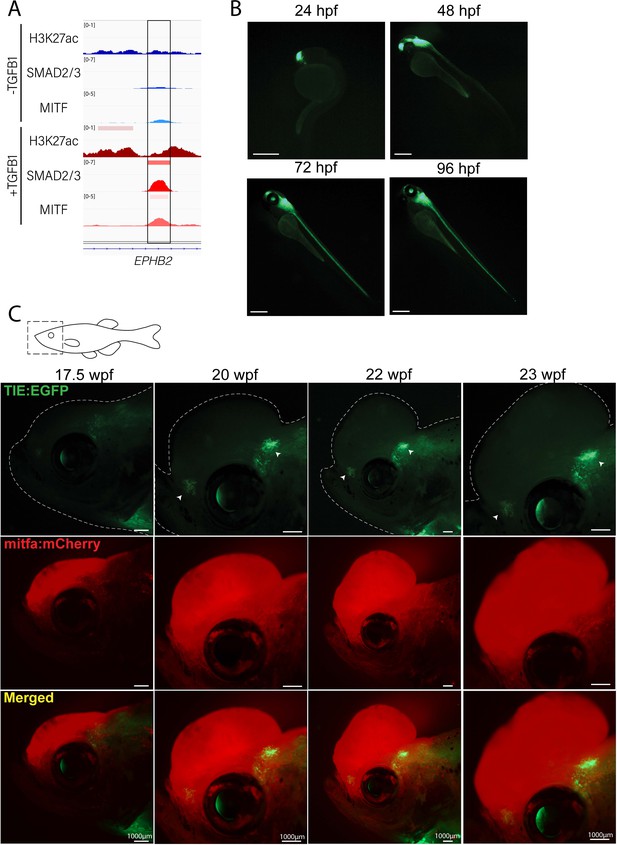
Novel TIE:EGFP zebrafish enhancer reporter is expressed in advanced melanomas.
(A) TGFb-induced enhancer (TIE) used to construct TIE:EGFP reporter determined by H3K27ac, SMAD2/3, and MITF ChIP-seq peaks in A375s+/-TGFB1. There is unique H3K27ac and SMAD2/3 binding upon stimulus. (B) TIE:EGFP expression throughout zebrafish development. Scale bars represent 500 µm. (C) TIE:EGFP expression across melanomagenesis indicated by arrowheads. Representative images shown. Additional tumors shown in Figure 1—figure supplement 3. Illustrated fish diagram in (C) created with BioRender.com, and published using a CC BY-NC-ND license with permission.
© 2024, BioRender Inc. Figure 1 was created using BioRender, and is published under a CC BY-NC-ND license. Further reproductions must adhere to the terms of this license.
The TIE:EGFP reporter construct was microinjected into Tg(mitfa:BRAFV600E);p53-/-;mitfa-/- zebrafish in order to generate melanomas. TIE:EGFP;Tg(mitfa:BRAFV600E);p53-/-;mitfa-/- stable embryos have EGFP expression in the anterior brain at 24 hr post fertilization (hpf) extending along the brain and spinal cord beginning at 48 hpf (Figure 1B). This expression pattern is consistent with that previously found in a Smad3 zebrafish reporter, although in our hands this Smad3 reporter was not active in adults (Casari et al., 2014). TIE:EGFP embryos were treated at 24 hpf with 50 or 100 µM of SB431542, a TGFb type I receptor kinase inhibitor, and imaged at 48 hpf (Kim et al., 2021). Treatment with this TGFb inhibitor for 24 hr drastically reduced TIE:EGFP signal (Figure 1—figure supplement 1B, C). This indicates that the TIE:EGFP reporter is specific to TGFb signaling.
TIE:EGFP reporter is expressed in advanced zebrafish melanomas
To visualize TGFb response across melanoma development, single cell Tg(mitfa:BRAFV600E);p53-/-;mitfa-/- embryos stably expressing TIE:EGFP were injected with an empty MiniCoopR vector (MCR:MCS) containing the mitfa minigene to generate melanomas. Tyrosinase gRNA and Cas9 protein were also injected to knock-out melanocyte pigment as well as mitfa:mCherry to allow for melanocyte visualization. Normal melanocytes rarely had TIE:EGFP expression, with the exception of occasional fin melanocytes (Figure 1—figure supplement 1D). TIE:EGFP has yet to be observed in mitfa:mCherry high early melanomas (Figure 1—figure supplement 1E). However, of the fish with melanoma formation (n=56), 55% turned on TIE:EGFP in advanced melanomas defined by protrusion from the body plane (Figure 1C). TIE:EGFP+ cells often occur in clusters throughout the tumor and these cells are TIE:EGFP+ for many weeks at a time. This data indicates that advanced zebrafish melanomas develop TGFb responsive zones.
TIE:EGFP expressing melanoma cells down-regulate interferon signaling
To understand the transcriptional differences between TIE:EGFP+ and TIE:EGFP- melanoma cells, single TIE:EGFP+ and mitfa:mCherry+ cells from TIE:EGFP expressing melanomas were processed for single cell RNA-sequencing at 23 and 42 weeks post fertilization (wpf), respectively (melanomas used are depicted in Figure 1C and Figure 1—figure supplement 2B). We used SORT-seq to sequence flow cytometry-sorted single cells and in silico linked transcriptomes to fluorescence intensities as recorded by FACS indexing (Muraro et al., 2016). A UMAP of the sequencing results from our combined replicates is shown (Figure 2A; UMAP with no batch correction in Figure 2—figure supplement 1A). Most cells were identified as melanoma cells expressing mitfa and/or sox10, but we identified an mpeg1.1-expressing TIE:EGFP+ macrophage cluster as well (Figure 2A).
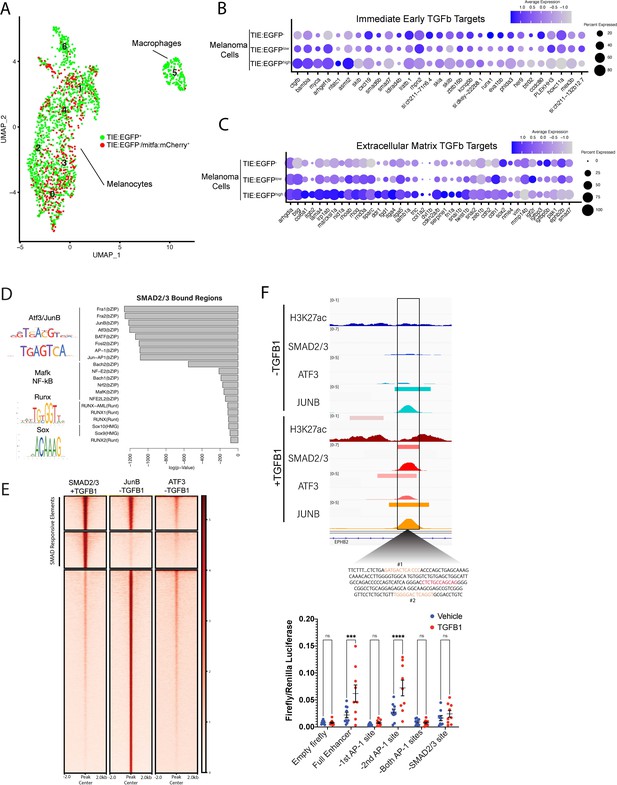
TGFb responsive melanoma cells in MCR:MCS tumors up-regulate chronic extracellular matrix TGFb target genes and AP-1 binding is required for TGFb responsiveness.
(A) (Left) UMAP depicting seven cell clusters identified by SORT-seq, combined two MCR:MCS biological replicates. Approximately 2256 TIE:EGFP+ cells and 752 mitfa:mCherry+;TIE:EGFP- cells (as a control) were sorted by flow cytometry for scRNA-seq. TIE:EGFP+ cells were both mitfa:mCherry+ and mitfa:mCherry-. In analysis of the scRNA-seq data, melanoma cells were identified as being mitfa and sox10 positive, while macrophages were identified as mpeg1.1 and marco positive. (B) Dotplot depicting TGFb immediate-early target gene expression in TIE:EGFPhigh, TIE:EGFPlow, and TIE:EGFP- melanoma cells. Melanoma cells can be segregated into TIE:EGFPhigh vs. TIE:EGFPlow based on EGFP intensity during sorting. (C) Dotplot depicting extracellular matrix TGFb target gene expression in TIE:EGFPhigh, TIE:EGFPlow, and TIE:EGFP- melanoma cells. (D) HOMER motif analysis of regulatory regions bound by SMAD2/3 upon stimulation in A375 cells. (E) Heatmap showing binding of JUNB and ATF3 pre-stimulus at 12,000 SMAD2/3-responsive elements in A375. (F) (Top) IGV plot of H3K27ac, SMAD2/3, ATF3 and JUNB ChIP-seq +/- TGFB1 stimulus at the TGFb-induced enhancer. Inset depicts sequence under SMAD2/3 ChIP-seq peak and highlights AP-1 (orange) and SMAD2/3 (red) binding sites. (Bottom) Firefly luciferase luminescence of full TIE reporter or reporter lacking AP-1 or SMAD2/3 sites. Normalized to Renilla transfection control. Experiment performed three times with three technical replicates each. A two-way multiple comparison ANOVA was used to calculate significance.
The melanoma cell population was separated into TIE:EGFP-, TIE:EGFPlow, and TIE:EGFPhigh based on FACS intensities. We found that immediate-early TGFb target genes, identified following an acute 2 hr TGFB1 treatment in zebrafish melanoma cells, ZMEL1, were down-regulated in TIE:EGFPhigh cells (Figure 2B). However, we identified 29 genes that were up-regulated in TIE:EGFPhigh cells. We termed these genes, related to the extracellular matrix, ‘chronic’ TGFb targets (Figure 2C; Katsuno et al., 2019; Verrecchia et al., 2001). At the time of dissociation, these melanomas had TIE:EGFP+ cells for several weeks, therefore they were likely not in an acute TGFb response phase (Figure 1C and Figure 1—figure supplement 2B). Using GSEA analysis we found that the top down-regulated pathways in TIE:EGFPhigh cells were interferon alpha and gamma (Figure 2—figure supplement 1B). This suggests that TGFb has an immune suppressive effect in these melanomas.
AP-1 factors are required for induction of the chronic TIE reporter
Although the vast majority of literature focuses on acute TGFb signaling, our TIE:EGFP reporter remains on in melanoma, reading out chronic TGFb signal. We next asked what transcription factors are necessary for induction of our novel chronic TGFb reporter and therefore required for chronic TGFb signaling. We performed HOMER motif analysis of ~12,000 SMAD2/3 responsive regulatory elements. These regions were identified in A375 melanoma cells stimulated with TGFB1 and were bound by SMAD2/3 upon stimulus. We found that these SMAD2/3 bound regions are highly enriched for AP-1 motifs (Figure 2D). ChIP-seq in A375 cells in the presence or absence of TGFB1 treatment confirmed the binding of AP-1 transcription factors JUNB and ATF3 (Figure 2E). ATF3 and JUNB were bound at 19% and 48% of SMAD2/3 responsive elements, respectively, before TGFB1 treatment was administered. This data indicates that AP-1 factors, in particular JUNB, may be important for SMAD binding to chromatin.
We hypothesized that AP-1 factors occupy SMAD responsive elements prior to stimulation, stabilizing open chromatin to allow the TGFb response to occur quickly upon stimulation. To test this hypothesis, we deleted AP-1 motifs in our TIE enhancer driving luciferase. We identified AP-1 motifs (highlighted in orange) in our TGFb-induced enhancer using MoLoTool and HOCOMOCO motifs (Figure 2F, top; Kulakovskiy et al., 2018). AP-1 motif #1 was the most significant (p=8.4e–6), and AP-1 motif #2 had a lesser p-value of 8.2e–4. We deleted either AP-1 site #1 alone, AP-1 site #2 alone, or both AP-1 sites, as well as the most significant SMAD2/3 motif (p=6.7e–6), shown in red (Figure 2—figure supplement 2). In A375 cells, loss of AP-1 site #1 rendered the enhancer region unresponsive to TGFb signaling, however loss of AP-1 site #2 did not alter inducibility (Figure 2F, bottom). This result indicates that AP-1 site #1 is necessary for TGFb inducibility at this enhancer. Deletion of the SMAD site also destroyed TGFB1 responsiveness. This data suggests that AP-1 binding is required for responsiveness of this chronic TGFb inducible enhancer in melanoma.
Macrophages preferentially phagocytose TIE:EGFP expressing melanoma cells
We identified TIE:EGFP+ macrophages in TIE:EGFP;Tg(mitfa:BRAFV600E);p53-/-;mitfa-/-;MCR:MCS melanomas that express mitfa and sox10, suggesting recent phagocytosis of melanoma cells (Figure 3A). There are two potential models for why macrophages are TIE:EGFP+: (1) macrophages express TIE:EGFP themselves, or (2) macrophages are engulfing TIE:EGFP+ cells. To test these hypotheses, we crossed TIE:EGFP;Tg(mitfa:BRAFV600E);p53-/-;mitfa-/- zebrafish to a mpeg:mCherry reporter line that labels macrophages with mCherry (Ellett et al., 2011). These embryos were injected with MCR:BRAFV600E, 2x U6:p53/Tyr gRNA mitfa:Cas9, and mitfa:BFP to generate pigmentless, BFP+ melanomas. As the TIE:EGFP reporter is cytoplasmic, if mpeg:mCherry+ macrophages are endogenously expressing TIE:EGFP, we would expect the entire macrophage to appear yellow via imaging. Confocal imaging of these tumors showed that the majority of a TIE:EGFP+ region of the tumor is composed of clustered TIE:EGFP+ macrophages. Within these regions, we observed that a subset of macrophages contain only puncta of TIE:EGFP signal, suggesting phagocytosis of TIE:EGFP+ cells, while a separate subset are entirely yellow (suggesting endogenous reporter expression; Figure 3B and Figure 3—figure supplement 1A). In 10 out of 13 tumors analyzed by confocal microscopy, about 60–80% of TIE:EGFP+ macrophages contained TIE:EGFP fragments, while the remaining TIE:EGFP+ macrophages were diffusely TIE:EGFP+. In the remaining three tumors, about 30% of TIE:EGFP+ macrophages contained TIE:EGFP fragments, while the remaining were diffusely TIE:EGFP+. Therefore, in most tumors (with rare exceptions), the majority of TIE:EGFP+ macrophages are EGFP+ because they phagocytosed a TIE:EGFP+ cell. Indeed, we observed macrophages actively phagocytosing TIE:EGFP+ cells (Figure 3—figure supplement 1C). In three out of 13 tumors imaged by confocal microscopy, we observed that in TIE:EGFP+ patches, macrophages were engaged closely with a cluster of TIE:EGFP+;mitfa:BFP+ tumor cells, likely in the process of phagocytosis (Figure 3B and Figure 3—figure supplement 1B). Together, this data reveals that a large proportion of macrophages engulf a TIE:EGFP+ cell population that includes melanoma cells.
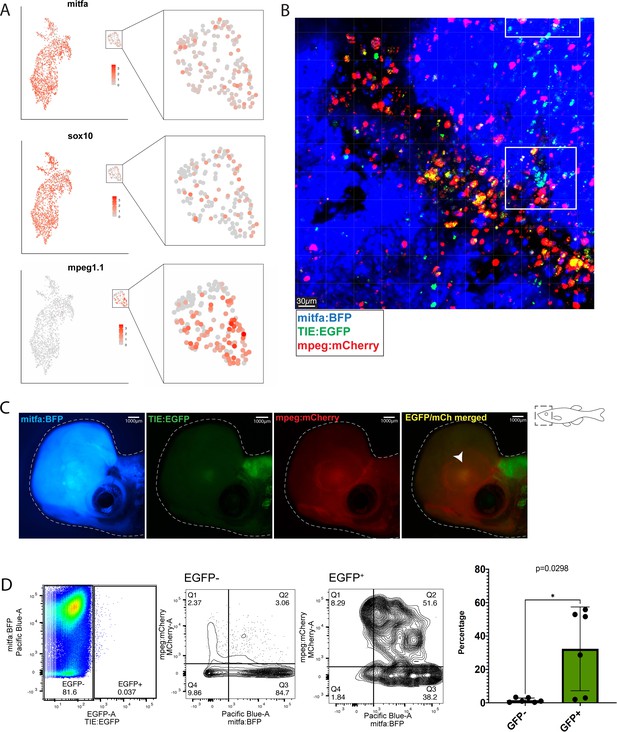
Macrophages preferentially phagocytose TIE:EGFP+ cells.
(A) UMAP depicting mitfa, sox10, and mpeg1.1 expression in clusters identified by SORT-seq, combined two MCR:MCS melanoma replicates. Inset shows expression of these genes in the macrophage cluster. (B) Representative image from a zebrafish melanoma acquired on an upright confocal, n=13 fish. Additional images shown in Figure 3—figure supplement 1. Melanoma cells are blue, macrophages are red, and TIE:EGFP+ cells are green. Yellow indicates a macrophage that has phagocytosed a TGFb responsive cell, which often appears as fragments within macrophages. A macrophage that expresses the TIE:EGFP endogenously would express EGFP throughout the entire cell, rather than in fragments. Cyan indicates a TIE:EGFP+ melanoma cell. When phagocytosed by macrophages, TIE:EGFP+ melanoma cells appear white, which are indicated within in the white boxes. (C) Representative TIE:EGFP+;mpeg:mCherry+;mitfa:BFP+ melanoma used for flow analysis of macrophages. Scale bars indicate 1000 µm. The EGFP+ region adjacent to the tumor is endogenous TIE:EGFP+ expression of the brain. (D) (Far left) Viable cells were separated into TIE:EGFP- and TIE:EGFP+. (Middle) FACS plots showing TIE:EGFP- and TIE:EGFP+ cells relative to mpeg:mCherry and mitfa:BFP. Q1 in the TIE:EGFP- plot represents macrophages that have not phagocytosed any melanoma cells. Q2 represents macrophages that have phagocytosed TIE:EGFP- melanoma cells. Q1 in the TIE:EGFP+ plot represents macrophages that have not phagocytosed melanoma cells, but rather express the TIE:EGFP reporter endogenously or phagocytosed a TIE:EGFP+ non-melanoma cell. Q2 represents macrophages that have phagocytosed TIE:EGFP+ melanoma cells. (Far right) Q2 of both plots are graphed to represent the percentage of all TIE:EGFP- or TIE:EGFP+ live cells that are melanoma cells phagocytosed by macrophages. Two-tailed unpaired Welch’s t-test was used to calculate significance. n=3 fish with two technical replicates each. Illustrated fish diagram in (C) created with BioRender.com, and published using a CC BY-NC-ND license with permission.
© 2024, BioRender Inc. Figure 3 was created using BioRender, and is published under a CC BY-NC-ND license. Further reproductions must adhere to the terms of this license.
To determine if macrophages are preferentially phagocytosing TIE:EGFP+ melanoma cells, three TIE:EGFP+;mpeg:mCherry+;mitfa:BFP+ melanoma were excised, digested, and processed for flow cytometry analysis (Figure 3C–D). Viable cells were separated into TIE:EGFP- and TIE:EGFP+ (Figure 3D, far left). In all three tumors, less than 1% of sorted cells were TIE:EGFP+, indicating this TGFb responsive population is rare. TIE:EGFP- and TIE:EGFP+ (Figure 3D, middle) cells were plotted relative to mpeg:mCherry and mitfa:BFP. Q2 represents the total percentage of TIE:EGFP- or TIE:EGFP+ cells that were melanoma cells phagocytosed by macrophages. The percentage given in Q2 is plotted for several tumors in Figure 3D (far right). Macrophages phagocytose a significantly higher percentage of TIE:EGFP+ melanoma cells compared to TIE:EGFP- melanoma cells. This was confirmed by qPCR, which showed that mitfa and sox10 expression is enriched in TIE:EGFP+ macrophages compared to TIE:EGFP- macrophages (Figure 3—figure supplement 2). Altogether, scRNA-seq, confocal imaging and flow cytometry analyses demonstrate that macrophages preferentially phagocytose TIE:EGFP+ melanoma cells compared to TIE:EGFP- melanoma cells.
SATB2 expression leads to early TGFb activation
The chromatin remodeler SATB2 is amplified in 4–8% of melanoma patients and its expression correlates with patient outcome. Our lab has previously shown that SATB2 overexpression leads to accelerated melanoma onset, produces more aggressive, metastatic tumors, and induces a TGFb signature (Fazio et al., 2021). SATB2 was overexpressed under the mitfa promoter in the MiniCoopR backbone (MCR:SATB2) in TIE:EGFP;Tg(mitfa:BRAFV600E);p53-/-;mitfa-/- zebrafish. Of the fish with SATB2 melanomas (n=27), 68% turned on TIE:EGFP in tumors, and its signal was expressed throughout the tumor volume (Figure 4A). To understand the transcriptional differences between TIE:EGFP+ and TIE:EGFP- melanoma cells, we sequenced the transcriptome of single cells from an MCR:SATB2 expressing melanoma at 30 wpf using SORT-seq (tumor used shown in Figure 4A, top). We identified most cells as melanoma cells with mitfa and/or sox10 expression, and again an mpeg1.1 expressing TIE:EGFP+ macrophage population (Figure 4B and Figure 4—figure supplement 1A).
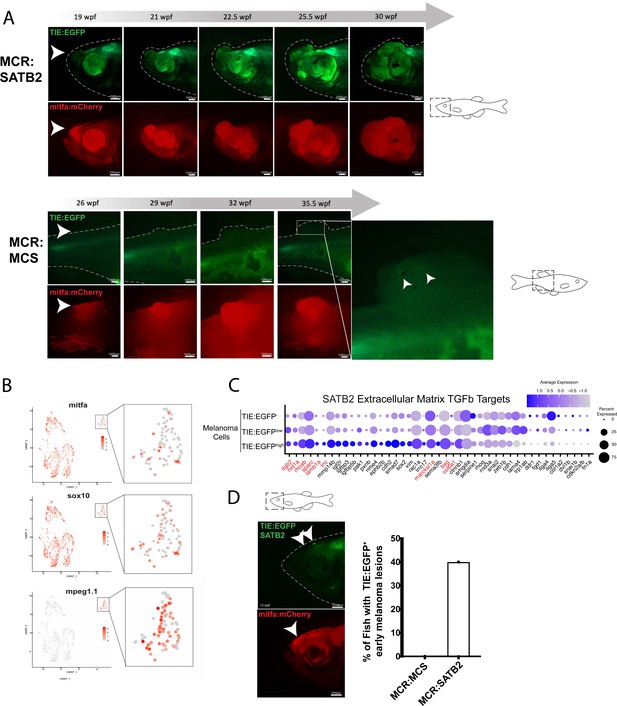
SATB2 expressing melanomas exhibit TIE:EGFP expression in early initiating lesions.
(A) (Top) Development of a representative tumor overexpressing MCR:SATB2 in TIE:EGFP;Tg(mitfa:BRAFV600E);p53-/-;mitfa-/- zebrafish. Arrowhead indicates TIE:EGFP+ early melanoma before tumor formation. This EGFP+ region is separate from the endogenous TIE:EGFP+ expression of the brain. (Bottom) Development of a control MCR:MCS tumor in TIE:EGFP;Tg(mitfa:BRAFV600E);p53-/-;mitfa-/- zebrafish, for reference. (B) UMAP depicting mitfa, sox10, and mpeg1.1 expression in clusters identified by SORT-seq of SATB2 expressing tumor in (A). Inset shows expression of these genes in the macrophage cluster. (C) Dotplot depicting extracellular matrix TGFb target gene expression in TIE:EGFPhigh, TIE:EGFPlow, and TIE:EGFP- SATB2 expressing melanoma cells. Genes shown in red are genes that are also upregulated in control MCR:MCS tumors. (D) Early initiating melanoma (arrowhead) overexpressing MCR:SATB2 in TIE:EGFP;Tg(mitfa:BRAFV600E);p53-/-;mitfa-/- zebrafish. Representative image chosen. 40% of MCR:SATB2 early melanomas (n=27) express TIE:EGFP, compared to 0% of MCR:MCS early melanomas (n=56) (quantification on right). Illustrated fish diagrams in (A, D) created with BioRender.com, and published using a CC BY-NC-ND license with permission.
© 2024, BioRender Inc. Figure 4 was created using BioRender, and is published under a CC BY-NC-ND license. Further reproductions must adhere to the terms of this license.
To determine if there is a change in state of the macrophages that express TIE:EGFP (either endogenously or via phagocytosis), we subset macrophages from the SATB2 expressing melanoma and separated macrophages that were TIE:EGFP+ or TIE:EGFP- based on flow cytometry intensity. TGFb is known to convert macrophages from a pro-inflammatory M1-like to an anti-inflammatory M2-like state which can be characterized by gene expression. In the zebrafish, these include M1 markers acod1, tnfa, csf3a/b, and socs3b, as well as M2 markers mrc1b, vegfaa, alox5ap, and marco (Viola et al., 2019; Wu et al., 2015; Jeannin et al., 2018; Wilson, 2014; Martinez and Gordon, 2014; Hwang et al., 2020; Ye et al., 2021; Georgoudaki et al., 2016). We found that although TIE:EGFP+ macrophages are not clearly polarized, they express a combination of M1-like and M2-like marker genes (Figure 4—figure supplement 1B).
To understand the transcriptional differences between TIE:EGFP+ and TIE:EGFP- melanoma cells, SATB2 expressing melanoma cells were divided into TIE:EGFP-, TIE:EGFPlow, and TIE:EGFPhigh cells based on flow cytometry intensity. These MCR:SATB2 results confirmed what was observed in the TIE:EGFP+ MCR:MCS tumors. We found that in TIE:EGFPhigh melanoma cells, chronic extracellular matrix TGFb target genes were up-regulated and the top down-regulated pathways by GSEA again were interferon alpha and gamma (Figure 4C and Figure 4—figure supplement 1C). Down-regulation of interferon suggests that the TGFb responsive melanoma cells likely evade adaptive immune responses, such as interferon-mediated antigen presentation to CD8+ T cells. Finally, 40% of the MCR:SATB2 fish with melanomas (n=27) had TIE:EGFP expressed in early melanomas. These lesions are mitfa:mCherry high, indicating a proliferation of melanoma cells, but do not yet extend off the body plane. Meanwhile, 0% of MCR:MCS fish with melanomas (n=56) had TIE:EGFP+ early melanomas (Figure 4D). This shows that overexpression of SATB2 leads to acceleration of TGFb response in melanoma and suggests patients with this amplification may be more susceptible to TGFb inhibitors.
Discussion
Here, we identified an inducible and specific TGFb enhancer reporter and visualized TGFb response throughout melanomagenesis in zebrafish. TIE:EGFP is off in early melanoma but is expressed in most advanced melanomas, and remains on, reading out chronic TGFb signaling. These TGFb responsive melanoma cells down-regulate interferon, up-regulate a series of novel chronic TGFb target genes, and are preferentially phagocytosed by macrophages. This work identifies a TGFb induced immune response and novel biomarkers of chronic TGFb signaling in melanoma.
We have shown that our reporter, generated from human ChIP-seq data, is able to read out chronic TGFb signaling. Most literature on developmental signaling focuses on acute signaling with stimulus for several hours in vitro. There are multiple explanations for why TIE:EGFP is a chronic TGFb reporter and once turned on, remains activated. Once the TIE:EGFP reporter is activated, it may remain active because chromatin is held in an open state by epigenetic modifications or AP-1 factors. We showed that AP-1 factors are necessary for TGFb induction of our TIE reporter and luciferase assays indicated that loss of AP-1 motifs eliminates basal levels of reporter transcription. AP-1 factors may be holding TIE chromatin open, therefore potentiating its ability to drive downstream reporter expression. Simultaneously, AP-1 factors likely hold chromatin open for other TGFb target genes to potentiate the TGFb response. This may allow for a rapid signaling response upon TGFb activation and suggests that AP-1 inhibitors could disrupt TGFb induction.
The TIE:EGFP scRNA-seq data indicates that melanoma cells responding to TGFb for several weeks see down-regulation of acute TGFb target genes. They exhibit up-regulation of 29 chronic TGFb target genes that are more representative of the downstream phenotypes imposed by TGFb signal, such as extracellular matrix genes. Based on the literature, these targets degrade extracellular matrix, promoting migration (Verrecchia et al., 2001). Some of the chronic TGFb targets were identified in one of the few reports of long-term TGFb signaling in human mammary epithelial cells for 12 or 24 days (Figures 2C and 4C). Prolonged TGFb treatment was found to stabilize EMT, stem cell state, and drug resistance in breast cancer cells (Katsuno et al., 2019). According to TCGA data in cBioPortal, a subset of melanoma patients exhibit up-regulation of these genes (Cerami et al., 2012; Gao et al., 2013). In the future, this signature could be used as biomarkers to identify patients with chronic TGFb signaling and these patients may benefit from treatment with TGFb inhibitors.
Overexpression of SATB2 resulted in TIE:EGFP expression in early melanoma lesions, which was not observed in controls. Cancer cells are thought to circumvent the tumor suppressive effects of TGFb via mutations or epigenetic modifications (Derynck et al., 2021; Kim et al., 2021; Massagué et al., 2000; Zhang et al., 2017; Seoane and Gomis, 2017; Suriyamurthy et al., 2019; Miyazawa and Miyazono, 2017; Tang et al., 2018). It is possible that melanomas up-regulating the epigenetic regulator SATB2 may overcome the tumor-suppressive effects of TGFb signaling earlier in tumor development, leading to more aggressive and invasive melanomas (Fazio et al., 2021). Investigating the activation of TGFb signaling in the context of different patient mutations may provide insight into who may benefit from TGFb inhibitors, allowing for the identification of biomarkers of TGFb inhibitor response. It is expected that aggressive melanoma subtypes like SATB2, which activate TGFb very early in melanoma development, would be most responsive to early intervention with TGFb inhibitors.
TIE:EGFP expression often occurs in patches throughout the tumor. In the tumor pictured in Figure 3C, there is general co-localization of mpeg:mCherry and TIE:EGFP signal indicating that macrophages may cluster in TGFb positive regions of the tumor. Using confocal imaging, we confirmed that TIE:EGFP signal is most often found in macrophages clustered in these zones. The TIE:EGFP reporter appears in some macrophages as a diffuse, cytoplasmic signal indicative of endogenous TGFb signaling. However in the majority of EGFP+ macrophages, the reporter appears as fragments (sometimes even in endosome-like structures), indicating that many macrophages are TIE:EGFP+ because they phagocytosed a TIE:EGFP+ cell. We occasionally captured through confocal imaging macrophages in contact with TIE:EGFP+ melanoma cells, suggesting that macrophages phagocytose these tumor cell populations (Figure 3—figure supplement 1). Indeed, our scRNA-seq data identified TIE:EGFP+ macrophage subclusters that express mitfa and/or sox10, providing additional evidence for phagocytosis of melanoma cells. Using flow cytometry analysis, we showed that macrophages preferentially phagocytose TIE:EGFP+ melanoma cells. The regionality of TGFb response could occur due to gradients in the TGFb morphogen, possibly induced by TGFb signaling within tumor cells in particular regions of the tumor. As mentioned above, TGFb can act as a chemoattractant for macrophages and induces an anti-inflammatory M2-like state. Once macrophages arrive to the TGFb region, they may phagocytose TIE:EGFP+ tumor cells, thus explaining the presence of EGFP+ fragments within macrophages. This data suggests that local TGFb signaling within the tumor may influence macrophage localization and phagocytosis, likely leading to changes in their behavior.
One factor we identified in our single cell RNA-seq data that may mediate this interaction is serpine1. Serpine1 mRNA was differentially upregulated in TIE:EGFP+ melanoma cells, and its receptor, lrp1ab was expressed on TIE:EGFP+ macrophages (data not shown). SERPINE1, which encodes plasminogen activator inhibitor-1 (PAI-1), has been shown to promote cancer cell invasiveness and macrophage recruitment in an esophageal squamous cell carcinoma model (Sakamoto et al., 2021). TIE:EGFPhigh macrophages differentially express mmp14b and the cysteine protease legumain, both of which have been described to promote TGFb bioavailability (Bai et al., 2019; Sounni et al., 2010; Robertson and Rifkin, 2016). Together, these preliminary transcriptional data may indicate a mechanism by which macrophages are recruited to TIE:EGFP+ regions by PAI-1 gradients. Recruited macrophages may phagocytose TIE:EGFP+ tumor cells and then go on to amplify the pool of active, bioavailable TGFb through the activity of enzymes such as mmp14b and lgmn. Such a model would explain the local clustering of macrophages in TGFb-responding regions of the tumor, where a subset phagocytoses TIE:EGFP+ melanoma and non-melanoma cells and others experience endogenous TGFb signaling (likely from local paracrine signaling).
Single cell RNA-seq data from the MCR:SATB2 melanoma suggests that TIE:EGFP+ macrophages (which are either TGFb responsive themselves or had phagocytosed a TGFb responding cell) are not clearly polarized, expressing transcriptional markers of both M1 and M2 states. This may indicate that a transition is occurring from the M1-like to the M2-like phenotype. In our model, macrophages are attracted to TGFb expressing regions of the melanoma, and while phagocytosing in the vicinity of TGFb cytokines, begin to transition to an M2 state. M2 macrophages are known to be anti-inflammatory, immunosuppressive, and pro-angiogenic. Preliminary evidence comparing the transcriptional signatures of macrophages in the SATB2 expressing tumor indicates that TIE:EGFP+ macrophages have higher expression of cholesterol and fatty acid genes (i.e. abca1a, npc2) as well as apoptotic genes (i.e. casp3b, caspa) compared to TIE:EGFP- macrophages. This indicates that intrinsic TGFb signaling or phagocytosis of TIE:EGFP+ cells may induce a stress phenotype within macrophages, resulting in death. In this case, death of macrophages over time would allow the tumor cells to evade phagocytosis. This, in conjunction with down-regulation of interferon by TIE:EGFP+ melanoma cells (as seen in MCR:MCS and MCR:SATB2 tumors), may lead to immune inactivation within the melanoma. Moreover, the interferon target gene, β–2-microglobulin (b2m) is one of the top down-regulated genes in TIE:EGFP expressing melanoma cells (Figure 2—figure supplement 1B). Loss of B2M, part of the MHC Class I molecules, is a known mechanism of immunotherapy resistance (Alavi et al., 2018). Together, this data suggests that activation of TGFb in melanoma is immunosuppressive, potentially by way of interferon modulation and effects on macrophage behavior and supports the need for more work on combination TGFb and immune checkpoint inhibitors.
Methods
Plasmids
Tgfb-Induced-Enhancer:Beta-globin-minimal-promoter-EGFP:pA pDestTol2pA2 was cloned by PCR amplifying the enhancer (chr1:22747452–22747734) in Figure 1A using A375 human melanoma gDNA (Forward primer: TTCTTTGTCATCCTGGTAGAGCAAATCGAG, Reverse primer: GACAGGTCGCACCTGAGTCC) (Advantage 2 PCR Kit, Clontech #639207, Kyoto, Japan). PCR product was gel purified (Qiagen #28604, Hilden, Germany) and cloned into a pENTR-5’TOPO vector (Invitrogen #45–0711, Waltham, MA, USA). This 5 ’entry vector was Gateway recombined upstream of a mouse beta-globin-minimal-promoter-EGFP middle entry vector, a 3 ’entry polyA, and the pDestTol2pA2 backbone (ThermoFisher #12538120, Waltham, MA, USA). Mitfa:mCherry was Gateway recombined using the mitfa zebrafish promoter and a mCherry middle entry plasmid (ThermoFisher #12538120). Ubi:caSMAD2 was cloned by PCR amplifying human SMAD2 pDONR221 (DNASU HsCD00045549, Tempe, AZ, USA) using the following primers to insert constitutively active mutations (Forward: CACCATGTCGTCCATCT TGCCATTCACGCCGCC;Reverse: CTATTCCATTTCTGAGCAACGCACTGAAGGGGATC) (Sigma 11732641001, St. Louis, MO, USA), gel purifying (Qiagen #28604) and inserting into a pENTR/D-TOPO vector (Invitrogen #45–0218). This middle entry vector was Gateway recombined using a 5’ entry zebrafish ubiquitious promoter (Addgene #2732, Watertown, MA, USA), a 3’ entry pA vector, and a pDestTol2pA2 backbone (ThermoFisher #12538120). Ubi:caSMAD3 was cloned by PCR amplifying human constitutively active SMAD3 from Smad3 pCMV-SPORT6 (Harvard Plasmid Repository #HSCD00339271, Boston, MA, USA) (Sigma 11732641001), gel purifying (Qiagen #28604) and inserting into a pENTR/D- TOPO vector (Invitrogen #45–0218). This middle entry vector was Gateway recombined using a 5’ entry zebrafish ubiquitious promoter, a 3’ entry pA vector, and a pDestTol2pA2 backbone (ThermoFisher #12538120). Ubi:BFP was cloned via Gateway recombination using a 5’ entry zebrafish ubiquitious promoter, a 3’ entry pA vector, and a pDestTol2pA2 backbone (ThermoFisher #12538120). Mitfa:BFP was cloned via Gateway recombination using a 5’ entry zebrafish mitfa promoter, a 3’ entry pA vector, and a pDestTol2pA2 backbone (ThermoFisher #12538120). MCR:SATB2 and MCR:MCS are from Fazio et al., 2021. MCR:BRAFV600E (Addgene #118846) and 2xU6:p53/tyr gRNA mitfa:Cas9 (Addgene #118844) are published (tyr gRNA: GGACTGGAGGACTTCTGGGG; p53 gRNA = GGTGGGAGAGTGGATGG CTG) (Ablain et al., 2018).
Reporter line creation
TIE:EGFP reporter fish were created by injecting TIE:Beta-globin-minimal-promoter- EGFP:pA pDestTol2pA2 at 6 ng/µL into single cell Tg(mitfa:BRAFV600E);p53-/-;mitfa-/- zebrafish embryos (referred to as TIE:EGFP;Tg(mitfa:BRAFV600E);p53-/-;mitfa-/-) (Patton et al., 2005). F0s were screened at 5 dpf for EGFP. At approximately 2 months post fertilization, F0s were crossed to Tg(mitfa:BRAFV600E);p53-/-;mitfa-/- fish and F1s were screened as embryos for EGFP. EGFP+ fish were raised to adulthood.
Electroporation
Electroporation protocols were adapted from Callahan et al., 2018. Tg(mitfa:BRAFV600E);p53-/-;mitfa-/- zebrafish were anesthetized using 4% MS-222 (Pentair, TRS1, Minneapolis, MN, USA). Zebrafish were subcutaneously injected with 2 µL mix using a Hamilton syringe (Hamilton #80300, Reno, NV, USA) anterior to the dorsal fin. The following amounts of vectors were injected into each fish: 200 ng TIE:Beta-globin-minimal-promoter-EGFP:pA pDestTol2pA2, 400 ng ubi:caSMAD2, 400 ng ubi:caSMAD3, 666 ng ubi:BFP, and 266 ng Tol2 pCS2FA-transposase (Kwan et al., 2007). DNA was prepped using Qiagen HiSpeed Plasmid Maxi Kit (QIAGEN #12662). Zebrafish were then electroporated with a BTX ECM 830 machine (BTX #45–0662 Holliston, MA, USA) using the following parameters: LV Mode; 45 V; 60ms pulse length; 5 pulses, 1 second interval. The cathode paddle was placed on the side of the fish that was injected. Electroporated fish were imaged approximately one week post electroporation using a Nikon SMZ18 Stereomicroscope (Nikon, Tokyo, Japan). To quantify TIE activity, we imaged each electroporated fish, quantified GFP intensity, and divided by the area of BFP to account for variation in electroporation efficiency.
Stereomicroscope and confocal imaging
For stereoscope imaging, zebrafish were anesthetized using 4% MS-222 (Pentair, TRS1) and imaged using a Nikon SMZ18 Stereomicroscope using consistent imaging parameters. For confocal imaging, tumors represented in Figure 3B and Figure 3—figure supplement 1C tumors were imaged using Nikon C2si Laser Scanning confocal using a 10 X objective at 1 X magnification. Z-stacks were aligned, and images minimally processed using Imaris (RRID:SCR_007370) or ImageJ software (RRID:SCR_003070). TIE:EGFP+ tumors depicted in Figure 3—figure supplement 1A, B were imaged using Zeiss LSM 980 NLO Multi-photon microscope using confocal detection at 15 X (Figure 3—figure supplement 1A, left), 45 X (Figure 3—figure supplement 1A, right), 20 X (Figure 3—figure supplement 1B). Z-stacks were aligned, and images were minimally processed using Imaris.
Inhibitor treatment
TIE:EGFP;Tg(mitfa:BRAFV600E);p53-/-;mitfa-/- F1s were crossed and F2 embryos were screened for EGFP, dechorionated at 24 hpf, and placed in a 24 well plate. Embryos were treated with either E3 zebrafish water, DMSO vehicle control (Sigma #D2650), 50 µM or 100 µM SB 431542 hydrate (Sigma # S4317-5MG). Twenty-four hours post treatment embryos were imaged using a Nikon SMZ18 Stereomicroscope (1 x objective; 3 x zoom; 7ms white light and 300ms GFP exposure used for all conditions).
In situ hybridization
SB-treated 48 hpf embryos (TIE:EGFP) were fixed with 4% PFA overnight at 4°C. The embryos were then dehydrated by washes of 1:2 MeOH:PBST, then 2:1 MeOH:PBST, then 100% MeOH, and stored in 100% MeOH. Embryos were re-hydrated using washes of 2:1 MeOH:PBT, then 1:2 MeOH:PBT, then 100% PBT. Samples were then digested with Proteinase K (Sigma Aldrich, #03115828001) at 10 µg/mL and washed with PBT. Samples were then re-fixed in 4% PFA, followed by several PBT washes. Samples were incubated in ‘PreHyb’ solution (50% formamide, 5 X SSC, 0.1% Tween 20, citric acid to pH6, 50 µg/mL heparin, 500 µg/mL tRNA) for 4.5 hr at 70 °C. They were then incubated in ‘ProbeHyb’ solution (same as PreHyb with addition of GFP1 probe at final concentration of 200 ng/500 µL) overnight at 70 °C. Samples were then consecutively washed in ‘WashHyb’ solution (50% formamide, 5 X SSC, 0.1% Tween 20, citric acid to pH6) at concentrations of 75%, then 50%, then 25% (in 2 X SSC) at 70 °C. Samples were then washed in 2 X SSC, then 0.2 X SSC (75%, then 50%, then 25%) in PBT. They were then incubated in blocking solution (PBT, 2% sheep serum, 2 mg/mL BSA) for 4 hr, then incubated in antibody solution (1:4000 of anti-DIG-AP (Sigma Aldrich, #11093274910) in blocking solution) overnight at 4 °C. Samples then underwent multiple PBT washes, then washing with staining wash solution (100 mM Tris HCL pH9.5, 50 mM MgCl2, 100 mM NaCl, 0.1% Tween 20). Samples were then stained (NBT 50 mg/mL, BCIP 50 mg/mL) for 30 min, then washed with PBT. Samples were post-fixed using 4% PFA at 4 °C overnight. Samples were then cleared using a series of MeOH washes: 1:2 MeOH:PBT, 2:1 MeOH:PBT, 100% MeOH. Samples were then washed with a series of glycerol washes: 30% glycerol (in PBT), 50% glycerol, 70% glycerol. They were then imaged in 70% glycerol using a stereomicroscope. Intensity of probe staining was analyzed, and embryos were binned into categories representing ‘low’, ‘medium’, or ‘high’, GFP intensity.
Melanoma generation
Melanocyte development is conserved between zebrafish and mammals (Mort et al., 2015). The master regulator of the melanocyte lineage, MITF, is conserved in zebrafish (mitfa) and required for melanocyte development (Lister et al., 1999). Expression of mitfa:BRAFV600E together with a homozygous p53 missense mutation, leads to development of zebrafish melanomas (Patton et al., 2005). A mitfa−/− mutation in Tg(mitfa:BRAFV600E);p53−/− fish prevents melanocyte development and spontaneous melanoma formation. Melanocyte development can then be mosaically rescued via injection of MiniCoopR (MCR), a transposon-based vector that contains a mitfa minigene, often alongside a candidate oncogene driven by the mitfa promoter (Ceol et al., 2011). In the absence of a candidate oncogene, the mitfa promoter is followed by an empty multiple cloning site (MCR:MCS), which is sufficient to generate melanomas in a genetic background already containing the BRAFV600E and p53-/- mutations. Tyrosinase, an enzyme required for melanocyte pigmentation, is also knocked out in our model to generate unpigmented melanomas, allowing for easy imaging of fluorescent reporters. TIE:EGFP;Tg(mitfa:BRAFV600E);p53-/- tumors were generated by injecting single cell TIE:EGFP;Tg(mitfa:BRAFV600E);p53-/-;mitfa-/- zebrafish embryos with MiniCoopR Multiple Cloning Site (MCR:MCS) at 20 ng/µL, mitfa:mCherry at 10 ng/µL, tyr gRNA (GGACTGGAGGACTTCTGGGG) at 10 ng/µL, Cas9 protein (PNA Bio CP02) at 50 ng/µL, and Tol2 mRNA at 20 ng/µL (White et al., 2008). Experiments involving the mpeg:mCherry reporter line required us to cross the TIE:EGFP;Tg(mitfa:BRAFV600E);p53-/- zebrafish line with a Casper (roy-/-;mitfa-/-) mpeg:mCherry line, so progeny would be heterozygous for the BRAFV600E and p53-/- mutations. We therefore generated TIE:EGFP;mpeg:mCherry;Tg(mitfa:BRAFV600E);p53-/- tumors by injecting into the one-cell stage MCR:BRAFV600E at 10 ng/µL, 2x U6:p53/Tyr gRNA mitfa:Cas9 at 10 ng/µL, mitfa:BFP at 10 ng/µL, tyr gRNA at 10 ng/µL, Cas9 protein at 50 ng/µL, and Tol2 mRNA at 20 ng/µL. Tyrosinase gRNA was created by annealing a tyr oligo template (CCTCCATACGATTTAGGTGACACTATAGGACTGGAGGACTTCTGGGGGTTTTAGAG CTAGAAATAGCAAG) to a constant oligonucleotide (AAAAGCACCGACTCGGTGCCACTT TTTCAAGTTGATAACGGACTAGCCTTATTTTAACTTGCTATTTCTAGCTCTAAAAC). The annealed oligo was filled in using T4 DNA polymerase (New England BioLabs, M0203S, Ipswich, MA, USA), PCR amplified, gel purified, transcribed (MEGAscript T7/SP6 Thermo Fisher Scientific, AM1333), and cleaned up (Zymo Research, R2051, Irvine, CA, USA). Embryos were grown to adulthood and were monitored for tumor development around 8–12 wpf.
Zebrafish work
All zebrafish (Danio rerio) work was performed in accordance with the Guide for the Care and Use of Laboratory Animals of the National Institutes of Health. Animal research protocols were approved by the Institutional Animal Care and Use Committee of Boston Children’s Hospital, Protocol #20-10-4254R. All zebrafish work operated according to the guidelines of the Institutional Animal Care and Use Committee of Boston Children’s Hospital.
SORT-seq
Tumors were excised and dissociated for 30 min with occasional chopping using 0.075 mg/mL Liberase (Sigma #5401119001) in DMEM (Gibco #11965–092, Waltham, MA, USA) with 1% Penstrep (Corning #30–002 CI, Corning, NY, USA). Casper zebrafish skin was used to exclude autofluorescence when setting gates (White et al., 2008). A mitfa:mCherry+/EGFP- tumor from a TIE:EGFP;mpeg:mCherry;Tg(mitfa:BRAFV600E);p53-/- fish or mitfa:mCherry;crestin:EGFP;tyr-/- zebrafish skin was used to set the gates for mCherry intensity. Ubi:EGFP zebrafish skin was used to set the gate for EGFP intensity. Dissociated samples were filtered through a 40 µm filter and resuspended in FACS buffer (PBS/10%FBS/1% Penstrep) before filtering through a FACS tube (Corning #352235). Single cells were sorted into 384-well-cell-capture plates containing barcoded primers from Single Cell Discoveries (https://www.scdiscoveries.com/) using a BD FACS ARIA II sorter. SYTOX was used as a live/dead marker (ThermoFisher #S34857). Library preparation and Illumina sequencing was performed by Single Cell Discoveries (Muraro et al., 2016). SORT-seq data are demultiplexed and aligned to zebrafish Ensembl GRCz11 annotation using scruff R packages with the following parameter (bcStart = 7, bcStop = 14, bcEdit = 1, umiStart = 1, umiStop = 6, keep = 60) (Hao et al., 2021, Wang et al., 2019). The 384 SORT-seq barcodes are downloaded from https://github.com/anna-alemany/transcriptomics/blob/master/mapandgo/bwamap/bc_celseq2.tsv ( Alemany, 2018). Analysis was completed in R Studio using Seurat (RRID:SCR_016341) (min.features=200; 600<nFeature RNA <10000; percent.mt <10; obj.resolution=0.2; GFPhigh>4000) (Hao et al., 2021; Stuart et al., 2019; Butler et al., 2018; Satija et al., 2015). Batch correction was performed using FindVariableFeatures and FindIntegrationAnchors, nfeatures = 15,000. Pathway analysis was conducted using Gene Set Enrichment Analysis (GSEA; RRID:SCR_003199) version 4.1.0 (Mootha et al., 2003; Subramanian et al., 2005). Zebrafish genes were converted to human using DIOPT Ortholog Finder version 8.5 (RRID:SCR_021963) and the best match was used for GSEA analysis (Hu et al., 2011).
Flow analysis
Tumors were excised and dissociated for 30 min with occasional chopping using 0.075 mg/mL Liberase (Sigma #5401119001) in DMEM (Gibco #11965–092) with 1% Penstrep (Corning #30–002 CI). Casper zebrafish skin was used to exclude autofluorescent cells (White et al., 2008). Mpeg:mCherry+ and ubi:EGFP+ zebrafish skin was used to set the gates for mCherry and EGFP intensity, respectively. A mitfa:BFP+ patch of skin as well as flk:BFP+ skin were used to set the gates for BFP intensity. Dissociated samples were filtered through a 40 µm filter and resuspended in FACS buffer (PBS/10%FBS/1% Penstrep) before filtering through a FACS tube (Corning #352235). DRAQ7 was used as a live/dead marker (Abcam #ab109202, Cambridge, UK). Cells were analyzed using a BD FACS Aria II 5 Laser System. Two technical replicates of 1 million cells were sorted from each tumor, for a total of 2 million cells per tumor. Data was processed using FlowJo version 10.8.1 (RRID:SCR_008520). Debris, doublets, and autofluorescent cells were removed from the analysis and viable cells were separated into TIE:EGFP- and TIE:EGFP+.
Human and zebrafish melanoma cell culture and treatment
Human melanoma A375 and zebrafish melanoma ZMEL1 cells were grown in filter sterilized DMEM (Gibco #11965–092) supplemented with 10% heat-inactivated FBS (Gemini # 900–108), 1% PenStrep (Corning #30–002 CI), and 1% Glutamine (ThermoFisher # 25030164). A375 cells were obtained from ATCC (RRID:CV-CL_0132) and grown at 37 °C, 5% CO2. Cell identity was confirmed by fingerprint every 2 years and tested for mycoplasma approximately every 2–4 weeks using Lonza’s second generation myco PLUS kit. ZMEL1 cells were grown at 28 °C, 5% CO2 and tested for mycoplasma approximately every 2–4 weeks (Heilmann et al., 2015). Human recombinant TGFB1 (R&D 240-B-002, Minneapolis, MN, USA) was reconstituted at 20 µg/mL in sterile 4 mM HCl containing 1 mg/mL BSA according to manufacturer’s instructions. To activate the TGFb pathway, cells were serum starved for 2 hr, then treated with 10 ng/mL human recombinant TGFB1 or 4 mM HCl containing 1 mg/mL BSA vehicle control for an additional 2 hr.
RNA-sequencing
RNA-seq was performed in triplicate using A375 or ZMEL1 melanoma cells. RNA was collected from adherent cells using the Qiagen RNeasy Plus Mini Kit (Qiagen #74134). RNA quality was confirmed using a Fragment Analyzer. One microgram of RNA was ribodepleted using NEBNext rRNA Depletion Kit (NEB #E6310). Ribodepleted RNA was fragmented, reverse transcribed, and library prepped (NEB #E7530, NEB #E7335). Samples were sequenced on an Illumina Hi-Seq 4000 sequencer. Quality control of RNA-Seq datasets was performed by FastQC (https://www.bioinformatics.babraham.ac.uk/projects/fastqc/) (RRID:SCR_014583) and Cutadapt (RRID:SCR_011841) to remove adaptor sequences and low quality regions (Martin, 2011). The high-quality reads were aligned to Ensembl build version GRCh38 of human genome or zebrafish Ensembl GRCz11 annotation (RRID:SCR_002334) using Tophat 2.0.11 (RRID:SCR_013035) without novel splicing form calls (Trapnell et al., 2009). Transcript abundance and differential expression were calculated with Cufflinks 2.2.1 (RRID:SCR_014597) (Trapnell et al., 2010). FPKM values were used to normalize and quantify each transcript; the resulting list of differentially expressed genes were filtered by log fold-change and q-value. Pathway analysis was conducted using Gene Set Enrichment Analysis (GSEA) version 4.1.0 Hallmark gene sets (Mootha et al., 2003; Subramanian et al., 2005). Deseq2 (RRID:SCR_015687) was used to create differential expression heatmaps and volcano plots. Read counts less than 10 were excluded.
ChIP-sequencing
A375 cells were fixed directly in 15 cm plates with 11% formaldehyde and collected by scraping. Approximately 100,000,000 cells were used per condition. Cells were lysed using lysis buffers with protease inhibitors (Roche #05056489001, Basel, Switzerland) and sonicated such that fragmented chromatin was 200–300 bp long. Optimal chromatin length was confirmed by gel electrophoresis. Prior to antibody addition, 50 µL chromatin was collected for input sample. The remaining sonicated chromatin was incubated overnight at 4 °C with 10 µg antibody attached to Dynabeads (Invitrogen #10004D). Samples (including inputs) were washed with wash buffers and eluted for 6 hr at 70 °C, treated with RNaseA (Sigma #R4642) and Proteinase K (Life Technologies #AM2546, Carlsbad, CA, USA), and purified using Zymo ChIP DNA Concentrator kit (Genesee Scientific #11–379 C, San Diego, CA, USA). Libraries were end repaired (VWR #ER81050, Radnor, PA, USA), polyA tailed (Invitrogen #18252–015, NEB #M0212L), adaptor ligated (NEB #E7335), size selected using Ampure XP beads (Beckman Coulter #A63881, Brea, CA, USA, Life Technologies #12027), and PCR amplified (NEB #M0531). Libraries were run on an Illumina Hi-Seq 4000 sequencer. The following antibodies were used: H3K27ac (Abcam #4729; RRID:AB_2118291), SMAD2/3 (Abcam #202445), MITF (Sigma #HPA003259; RRID:AB_1079381), ATF3 (Abcam #207434; RRID:AB_2734728), JUNB (CST #3753, Danvers, MA, USA; RRID:AB_2130002). Using HOMER analysis (RRID:SCR_010881) we confirmed that JUNB and ATF3 binding motifs were present under their respective ChIP peaks (ATF3, p=1e–4983) (JUNB, p=1e–15697). All ChIP-Seq datasets were aligned to Ensembl build version GRCh38 of the human genome using Bowtie2 (version 2.2.1; RRID:SCR_016368) with the following parameters: --end-to-end, -N0, -L2086. MACS2 version 2.1.0 (RRID:SCR_013291) peak finding algorithm was used to identify regions of ChIP-Seq peaks, with a q-value threshold of enrichment of 0.05 for all datasets (Langmead and Salzberg, 2012; Zhang et al., 2008). Uropa (Universal Robust Peak Annotator) is utilized to annotate ChIP-seq peaks to neighboring genes according to Ensembl gene annotation (Kondili et al., 2017). The parameters are defined as proximal promoter: 500 bp upstream – 50 bp downstream of TSS; distal promoter: 2 k bp upstream – 500 bp downstream of TSS; enhancer: 100 k bp from TSS. The genome-wide transcription factor SMAD2/3, JUNB, ATF3 occupancy profile figures were generated by deeptools2 according to two computation modes (Ramírez et al., 2016). In the reference-point mode, a set of genomic positions (e.g. the center of ChIP peak) are used as anchor point, 2 kb upstream and downstream of these position are plotted in the profile figure. HOMER analysis was performed to confirm transcription factor binding under peaks (Heinz et al., 2010). The hg19 genome was used with a random set of background peaks for motif enrichment.
Luciferase assays
Firefly luciferase reporter constructs (pGL4.24) were created by cloning the full and mutated TGFb enhancers upstream of the minimal promoter using BglII and XhoI sites (see Figure 2—figure supplement 2 for sequences). A375 cells were plated in opaque-walled 96-well plates (Thermo Fisher #136101) and approximately 5000 cells were co-transfected with 100 ng firefly and 10 ng Renilla luciferase plasmids using Lipofectamine 3000 (Invitrogen #L3000008). After 48 hr cells were serum starved for 2 hr and treated with 10 ng/mL TGFB1 or 4 mM HCl containing 1 mg/mL BSA vehicle control for an additional 2 hr. Firefly and Renilla luciferase were then measured using the Dual-Glo Luciferase Assay (Promega #E2920, Madison, WI, USA) according to the manufacturer’s instructions. Luminescence was read on a Synergy Neo plate reader and the ratio of firefly to Renilla luminescence was calculated. Empty firefly luciferase vector was used as a negative control and Renilla luciferase was used as control for transfection efficiency. Experiments were performed in biological triplicate with three technical replicates each.
qPCR
Two TIE:EGFP;mpeg:mCherry;Tg(mitfa:BRAFV600E);p53-/-;mitfa:BFP tumors were dissociated for 30 min with occasional chopping using 0.075 mg/mL Liberase (Sigma #5401119001) in DMEM/F12 (Gibco # 11580546), and the reaction was inactivated by adding a solution of 15% FBS in DMEM/F12. Dissociated samples were filtered through a 40 µm filter and resuspended in FACS buffer (PBS/5% BSA). Casper zebrafish skin was used to exclude autofluorescent cells, and mpeg:mCherry+ zebrafish skin was used to set the mCherry gate. SYTOX red dead cell stain was used as a live/dead marker (Invitrogen #S34859). Cells were sorted into FACS buffer in two separate tubes: mpeg:mCherry+ TIE:EGFP+ and mpeg:mCherry+ TIE:EGFP-. RNA was extracted from cells sorted by FACS using the Direct-zol RNA MicroPrep Kit (Zymo Research #2060), and reverse transcribed to cDNA using the SuperScript VILO cDNA Synthesis Kit (Invitrogen #11754–050). qPCR was performed using SYBR Green qPCR Mix (ThermoFisher # 4309155), and samples were run using the QuantStudio 6 Flex system (ThermoFisher). Mitfa primer sequences (FP: 5’CTACGACAGCCCAAACAAGG, RP: 5 ’GCCATTGTCATGTTCGTCCA). Sox10 primer sequences (FP: 5 ’ACGCTACAGGTCAGAGT CAC, RP: ATGTTGGCCATCACGTCATG). Data was analyzed using the delta delta Ct method. Ct values were normalized to those of housekeeping gene, b-actin. B-actin primer sequences (FP: CGAGCAGGAGATGGGAACC, RP: CAACGGAAACGCTCATTGC).
Statistics
To calculate significance of electroporation and flow analysis assays, a two-tailed unpaired t-test with Welch’s correction was performed using GraphPad Prism version 9.0.2 (RRID:SCR_002798) for Mac (GraphPad Software, San Diego, California USA, https://www.graphpad.com). To calculate significance of luciferase assays, a 2-way multiple comparison ANOVA test was performed using GraphPad Prism version 9.0.2 for Mac. For the qPCR experiment in Figure 3—figure supplement 2, 2-tailed unpaired t-tests was performed using GraphPad Prism for Mac.
Data availability
Sequencing datasets can be accessed on GEO at # GSE213360. The Tgfb-Induced-Enhancer:Beta-globin-minimal-promoter-EGFP:pA pDestTol2pA2 plasmid is available on Addgene (Plasmid #220508), and the TIE:EGFP zebrafish reporter line is available upon request.
-
NCBI Gene Expression OmnibusID GSE213360. A chronic signaling TGFb zebrafish reporter identifies immune response in melanoma.
References
-
Interferon signaling is frequently downregulated in melanomaFrontiers in Immunology 9:1414.https://doi.org/10.3389/fimmu.2018.01414
-
Induction of growth factor RNA expression in human malignant melanoma: markers of transformationCancer Research 51:4815–4820.
-
Rapid onset synovial inflammation and hyperplasia induced by transforming growth factor betaThe Journal of Experimental Medicine 171:231–247.https://doi.org/10.1084/jem.171.1.231
-
Macrophage-Derived Legumain Promotes Pulmonary Hypertension by Activating the MMP (Matrix Metalloproteinase)-2/TGF (Transforming Growth Factor)-β1 SignalingArteriosclerosis, Thrombosis, and Vascular Biology 39:e130–e145.https://doi.org/10.1161/ATVBAHA.118.312254
-
Cancer modeling by Transgene Electroporation in Adult Zebrafish (TEAZ)Disease Models & Mechanisms 11:dmm034561.https://doi.org/10.1242/dmm.034561
-
TGFβ biology in cancer progression and immunotherapyNature Reviews. Clinical Oncology 18:9–34.https://doi.org/10.1038/s41571-020-0403-1
-
Transforming growth factor-beta in cutaneous melanomaPigment Cell & Melanoma Research 21:123–132.https://doi.org/10.1111/j.1755-148X.2008.00450.x
-
Human monocytes and macrophages regulate immune tolerance via integrin αvβ8-mediated TGFβ activationThe Journal of Experimental Medicine 215:2725–2736.https://doi.org/10.1084/jem.20171491
-
Novel therapies emerging in oncology to target the TGF-β pathwayJournal of Hematology & Oncology 14:55.https://doi.org/10.1186/s13045-021-01053-x
-
Transforming growth factor beta inhibits the antigen-presenting functions and antitumor activity of dendritic cell vaccinesCancer Research 63:1860–1864.
-
UROPA: a tool for Universal RObust Peak AnnotationScientific Reports 7:2593.https://doi.org/10.1038/s41598-017-02464-y
-
The Tol2kit: A multisite gateway-based construction kit for Tol2 transposon transgenesis constructsDevelopmental Dynamics 236:3088–3099.https://doi.org/10.1002/dvdy.21343
-
Enhanced preclinical antitumor activity of M7824, a bifunctional fusion protein simultaneously targeting PD-L1 and TGF-βScience Translational Medicine 10:eaan5488.https://doi.org/10.1126/scitranslmed.aan5488
-
Fast gapped-read alignment with Bowtie 2Nature Methods 9:357–359.https://doi.org/10.1038/nmeth.1923
-
TGF-β-induced (TGFBI) protein in melanoma: A signature of high metastatic potentialThe Journal of Investigative Dermatology 134:1675–1685.https://doi.org/10.1038/jid.2014.20
-
Regulation of TGF-β Family Signaling by Inhibitory SmadsCold Spring Harbor Perspectives in Biology 9:a022095.https://doi.org/10.1101/cshperspect.a022095
-
The melanocyte lineage in development and diseaseDevelopment 142:620–632.https://doi.org/10.1242/dev.106567
-
Insights into the Transforming Growth Factor-β Signaling Pathway in Cutaneous MelanomaAnnals of Dermatology 25:135–144.https://doi.org/10.5021/ad.2013.25.2.135
-
deepTools2: a next generation web server for deep-sequencing data analysisNucleic Acids Research 44:W160–W165.https://doi.org/10.1093/nar/gkw257
-
Regulation of the Bioavailability of TGF-β and TGF-β-Related ProteinsCold Spring Harbor Perspectives in Biology 8:a021907.https://doi.org/10.1101/cshperspect.a021907
-
Constitutive expression of multiple growth factor genes by melanoma cells but not normal melanocytesThe Journal of Investigative Dermatology 97:20–26.https://doi.org/10.1111/1523-1747.ep12477822
-
Transforming growth factor beta production and responsiveness in normal human melanocytes and melanoma cellsCancer Research 54:575–581.
-
PAI-1 derived from cancer-associated fibroblasts in esophageal squamous cell carcinoma promotes the invasion of cancer cells and the migration of macrophagesLaboratory Investigation; a Journal of Technical Methods and Pathology 101:353–368.https://doi.org/10.1038/s41374-020-00512-2
-
Spatial reconstruction of single-cell gene expression dataNature Biotechnology 33:495–502.https://doi.org/10.1038/nbt.3192
-
TGF-β Family Signaling in Tumor Suppression and Cancer ProgressionCold Spring Harbor Perspectives in Biology 9:a022277.https://doi.org/10.1101/cshperspect.a022277
-
Stromal regulation of vessel stability by MMP14 and TGFbetaDisease Models & Mechanisms 3:317–332.https://doi.org/10.1242/dmm.003863
-
TopHat: discovering splice junctions with RNA-SeqBioinformatics 25:1105–1111.https://doi.org/10.1093/bioinformatics/btp120
-
Melanoma-associated expression of transforming growth factor-beta isoformsThe American Journal of Pathology 148:1887–1894.
-
Identification of novel TGF-beta /Smad gene targets in dermal fibroblasts using a combined cDNA microarray/promoter transactivation approachThe Journal of Biological Chemistry 276:17058–17062.https://doi.org/10.1074/jbc.M100754200
-
The metabolic signature of macrophage responsesFrontiers in Immunology 10:1462.https://doi.org/10.3389/fimmu.2019.01462
-
Nonclinical Development of SRK-181: An Anti-Latent TGFβ1 Monoclonal Antibody for the Treatment of Locally Advanced or Metastatic Solid TumorsInternational Journal of Toxicology 40:226–241.https://doi.org/10.1177/1091581821998945
-
SOCS Proteins in Macrophage Polarization and FunctionFrontiers in Immunology 5:357.https://doi.org/10.3389/fimmu.2014.00357
-
TNF-a mediated inflammatory macrophage polarization contributes to the pathogenesis of steroid-induced osteonecrosis in miceInternational Journal of Immunopathology and Pharmacology 28:351–361.https://doi.org/10.1177/0394632015593228
-
Model-based analysis of ChIP-Seq (MACS)Genome Biology 9:R137.https://doi.org/10.1186/gb-2008-9-9-r137
-
TGF-β Family Signaling in the Control of Cell Proliferation and SurvivalCold Spring Harbor Perspectives in Biology 9:a022145.https://doi.org/10.1101/cshperspect.a022145
Article and author information
Author details
Funding
National Institutes of Health (NCI R01CA103846)
- Haley R Noonan
- Alexandra M Thornock
- Julia Barbano
- Michael Xifaras
- Chloe S Baron
- Song Yang
- Katherine Koczirka
- Alicia M McConnell
- Leonard I Zon
Melanoma Research Alliance (Grant given via University of Edinburgh United Kingdom project number TBD)
- Alexandra M Thornock
- Michael Xifaras
- Chloe S Baron
- Song Yang
- Leonard I Zon
National Institutes of Health (P01CA163222)
- Haley R Noonan
- Alexandra M Thornock
- Julia Barbano
- Michael E Xifaras
- Chloe S Baron
- Song Yang
- Katherine Koczirka
- Alicia M McConnell
- Leonard I Zon
The funders had no role in study design, data collection and interpretation, or the decision to submit the work for publication.
Acknowledgements
We thank Christian Lawrence, Kara Maloney, Shane Hurley, Lauren McKay, Li- Kun Zhang, and Andrew Kowalczyk for fish care throughout this study. We also thank the Boston Children’s Hospital Flow Cytometry Research Facility for assistance with FACS experiments, as well as Single Cell Discoveries for processing SORT-seq samples.
We thank the Harvard Center for Biological Imaging (RRID:SCR_018673) for infrastructure and support. The authors thank their colleagues for discussion and reading of this manuscript. Figures were created with Biorender.com. Leonard I Zon is a Howard Hughes Medical Institute Investigator. Additional funding for this work was provided by the Human Frontier Science Program (HFSP LT000494/2020 L, to C.S.B.).
Ethics
All zebrafish (Danio rerio) work was performed in accordance with the Guide for the Care and Use of Laboratory Animals of the National Institutes of Health. Animal research protocols were approved by the Institutional Animal Care and Use Committee of Boston Children's Hospital, Protocol #20-10-4254R. All zebrafish work operated according to the guidelines of the Institutional Animal Care and Use Committee of Boston Children's Hospital.
Copyright
© 2024, Noonan, Thornock et al.
This article is distributed under the terms of the Creative Commons Attribution License, which permits unrestricted use and redistribution provided that the original author and source are credited.
Metrics
-
- 459
- views
-
- 43
- downloads
-
- 1
- citations
Views, downloads and citations are aggregated across all versions of this paper published by eLife.
Download links
Downloads (link to download the article as PDF)
Open citations (links to open the citations from this article in various online reference manager services)
Cite this article (links to download the citations from this article in formats compatible with various reference manager tools)
Further reading
-
- Cancer Biology
- Immunology and Inflammation
The immunosuppressive microenvironment in pancreatic ductal adenocarcinoma (PDAC) prevents tumor control and strategies to restore anti-cancer immunity (i.e. by increasing CD8 T-cell activity) have had limited success. Here, we demonstrate how inducing localized physical damage using ionizing radiation (IR) unmasks the benefit of immunotherapy by increasing tissue-resident natural killer (trNK) cells that support CD8 T activity. Our data confirms that targeting mouse orthotopic PDAC tumors with IR together with CCR5 inhibition and PD1 blockade reduces E-cadherin positive tumor cells by recruiting a hypoactive NKG2D-ve NK population, phenotypically reminiscent of trNK cells, that supports CD8 T-cell involvement. We show an equivalent population in human single-cell RNA sequencing (scRNA-seq) PDAC cohorts that represents immunomodulatory trNK cells that could similarly support CD8 T-cell levels in a cDC1-dependent manner. Importantly, a trNK signature associates with survival in PDAC and other solid malignancies revealing a potential beneficial role for trNK in improving adaptive anti-tumor responses and supporting CCR5 inhibitor (CCR5i)/αPD1 and IR-induced damage as a novel therapeutic approach.
-
- Cancer Biology
Clonal hematopoiesis of indeterminate potential (CHIP) allows estimation of clonal dynamics and documentation of somatic mutations in the hematopoietic system. Recent studies utilizing large cohorts of the general population and patients have revealed significant associations of CHIP burden with age and disease status, including in cancer and chronic diseases. An increasing number of cancer patients are treated with immune checkpoint inhibitors (ICIs), but the association of ICI response in non-small cell lung cancer (NSCLC) patients with CHIP burden remains to be determined. We collected blood samples from 100 metastatic NSCLC patients before and after ICI for high-depth sequencing of the CHIP panel and 63 samples for blood single-cell RNA sequencing. Whole exome sequencing was performed in an independent replication cohort of 180 patients. The impact of CHIP status on the immunotherapy response was not significant. However, metastatic lung cancer patients showed higher CHIP prevalence (44/100 for patients vs. 5/42 for controls; p = 0.01). In addition, lung squamous cell carcinoma (LUSC) patients showed increased burden of larger clones compared to lung adenocarcinoma (LUAD) patients (8/43 for LUSC vs. 2/50 for LUAD; p = 0.04). Furthermore, single-cell RNA-seq analysis of the matched patients showed significant enrichment of inflammatory pathways mediated by NF-κB in myeloid clusters of the severe CHIP group. Our findings suggest minimal involvement of CHIP mutation and clonal dynamics during immunotherapy but a possible role of CHIP as an indicator of immunologic response in NSCLC patients.