Cis-regulatory modes of Ultrabithorax inactivation in butterfly forewings
eLife assessment
This valuable paper examines the Bithorax complex in several butterfly species, in which the complex is contiguous and not split, as it is in the well-studied fruit fly Drosophila. Based on genetic screens and genetic manipulations of a boundary element involved in segment-specific regulation of Ubx, the authors provide convincing evidence for their conclusions, which could be strengthened by additional data and analyses in the future. The data presented are relevant for those interested in the evolution and function of Hox genes and of gene regulation in general.
https://doi.org/10.7554/eLife.90846.3.sa0Valuable: Findings that have theoretical or practical implications for a subfield
- Landmark
- Fundamental
- Important
- Valuable
- Useful
Convincing: Appropriate and validated methodology in line with current state-of-the-art
- Exceptional
- Compelling
- Convincing
- Solid
- Incomplete
- Inadequate
During the peer-review process the editor and reviewers write an eLife Assessment that summarises the significance of the findings reported in the article (on a scale ranging from landmark to useful) and the strength of the evidence (on a scale ranging from exceptional to inadequate). Learn more about eLife Assessments
Abstract
Hox gene clusters encode transcription factors that drive regional specialization during animal development: for example the Hox factor Ubx is expressed in the insect metathoracic (T3) wing appendages and differentiates them from T2 mesothoracic identities. Hox transcriptional regulation requires silencing activities that prevent spurious activation and regulatory crosstalks in the wrong tissues, but this has seldom been studied in insects other than Drosophila, which shows a derived Hox dislocation into two genomic clusters that disjoined Antennapedia (Antp) and Ultrabithorax (Ubx). Here, we investigated how Ubx is restricted to the hindwing in butterflies, amidst a contiguous Hox cluster. By analysing Hi-C and ATAC-seq data in the butterfly Junonia coenia, we show that a Topologically Associated Domain (TAD) maintains a hindwing-enriched profile of chromatin opening around Ubx. This TAD is bordered by a Boundary Element (BE) that separates it from a region of joined wing activity around the Antp locus. CRISPR mutational perturbation of this BE releases ectopic Ubx expression in forewings, inducing homeotic clones with hindwing identities. Further mutational interrogation of two non-coding RNA encoding regions and one putative cis-regulatory module within the Ubx TAD cause rare homeotic transformations in both directions, indicating the presence of both activating and repressing chromatin features. We also describe a series of spontaneous forewing homeotic phenotypes obtained in Heliconius butterflies, and discuss their possible mutational basis. By leveraging the extensive wing specialization found in butterflies, our initial exploration of Ubx regulation demonstrates the existence of silencing and insulating sequences that prevent its spurious expression in forewings.
Introduction
Hox genes are key specifiers of antero-posterior regional identity in animals, and thus require robust regulatory mechanisms that confine their expression to well-delimited sections of the body (Lewis, 1978). Their genomic arrangement into Hox gene clusters has provided a rich template for the study of gene regulation, with mechanisms including chromatin silencing and opening, 3D conformational changes, and non-coding RNAs (Mallo and Alonso, 2013). However, this rich body of work has been almost exclusively performed in mice and fruit flies. In order to decipher how diverse body plans and morphologies evolved, we must start assessing the mechanisms of Hox gene regulation in a wider range of organisms.
The Ultrabithorax (Ubx) gene encodes a Hox family transcription factor involved in the specification of segment identities in arthropods (Hughes and Kaufman, 2002; Heffer and Pick, 2013). In insects, the conserved expression of Ubx in the metathoracic (T3) segment is required for their differentiation from Ubx-free tissues in the mesothorax (T2), and has been a key factor for the specialization of metathoracic serial appendages including T3 legs (Mahfooz et al., 2007; Refki et al., 2014; Tomoyasu, 2017; Feng et al., 2022; Buffry et al., 2023) and hindwings or their derivatives (Tomoyasu, 2017; Loker et al., 2021). The mechanisms of Ubx segment-specific expression have been intensively studied in D. melanogaster (Mallo and Alonso, 2013; Hajirnis and Mishra, 2021), where Hox genes are separated into two genomic loci, the Antennapedia (ANT-C, Antp) and Bithorax clusters (BX-C). In short, the BX-C complex that includes Ubx, abdominal-A (abd-A), and Abdominal-B (Abd-B) is compartmentalized into nine chromosomal domains that determine the parasegmental expression boundaries of these three genes (Maeda and Karch, 2015). For instance, the deletion of a small region situated between Ubx and abd-A produces the Front-ultraabdominal phenotype (Fub) where the first abdominal segment (A1) is transformed into a copy of the second abdominal segment A2, due to a gain-of-expression of abd-A in A1 where it is normally repressed (Pease et al., 2013). At the molecular level, the Fub boundary is enforced by insulating factors that separate Topologically Associating Domains (TADs) of open-chromatin, while also allowing interactions of Ubx and abd-A enhancers with their target promoters (Postika et al., 2018; Srinivasan and Mishra, 2020). Likewise, the Fab-7 deletion, which removes a TAD boundary insulating abd-A and Abd–B (Moniot-Perron et al., 2023), transforms parasegment 11 into parasegment 12 due to an anterior gain-of-expression of Abd-B (Gyurkovics et al., 1990). By extrapolation, one may expect that if the Drosophila Hox locus was not dislocated into two complexes, Antp and Ubx 3D contact domains would be separated by a Boundary Element (BE), and that deletions similar with Fub and Fab-7 mutations would result in gain-of-function mutations of Ubx that could effectively transform T2 regions into T3 identities. The BX-C locus also includes non-coding RNAs (Pease et al., 2013), some of which are processed into miRNAs known to repress Ubx and abd-A (Gummalla et al., 2012; Garaulet and Lai, 2015). Fub-1/bxd long non-coding RNAs (lncRNAs) situated 5’ of Ubx are thought to participate in Ubx regulation in the PS5 (posterior T3 to anterior A1) parasegment (Petruk et al., 2006; Ibragimov et al., 2023). An intronic lncRNA dubbed lncRNA:PS4 is expressed in the PS4 parasegment (posterior T2 - anterior T3), and appears to stabilize Ubx in this region in mutant contexts (Hermann et al., 2022). Little is known about how insect Hox genes are regulated outside of Drosophila, where they co-localize into a single Hox cluster, and where Antp and Ubx thus occur in contiguous positions (Gaunt, 2022; Mulhair and Holland, 2024). A few Hox-related miRNAs are evolutionarily conserved across the locus in arthropods (Pace et al., 2016), and an early study in Tribolium characterized the embryonic expression of a Hox cluster non-coding transcripts (Shippy et al., 2008).
These knowledge gaps lead us to consider the use of butterflies and moths (Lepidoptera) as alternative model systems for the study of Ubx function and regulation. Lepidopteran forewings and hindwings are functionally and morphologically differentiated, and CRISPR mosaic knock-outs (mKOs) showed that Ubx is necessary for the specification of hindwing color patterns, shape, and venation (Tendolkar et al., 2021). In three species of nymphalid butterflies (Heliconius erato, Junonia coenia, and Bicyclus anynana), CRISPR-mediated loss-of-function of Ubx induces regional-specific homeotic transformations of hindwing patterns into their forewing counterpart (Matsuoka and Monteiro, 2018; Tendolkar et al., 2021), reminiscent of homeotic aberrations that are sporadically observed in butterfly wings (Sibatani, 1983; Nijhout and Rountree, 1995). The ectopic activation of Ubx into the pupal forewing results in the gain of hindwing features, suggesting Ubx is sufficient to drive T3-like identity when expressed in T2 (Lewis et al., 1999; Tong et al., 2014). Besides its roles in adult wing differentiation, Ubx is also known to repress thoracic leg identity in transient embryonic appendages of the first abdominal segment, called pleuropods (Zheng et al., 1999; Masumoto et al., 2009; Tong et al., 2017; Tendolkar et al., 2021; Matsuoka et al., 2022). The general organization of Hox gene clusters has been well described in Lepidoptera, but their regulation has been seldom studied. Lepidopteran genomes have accumulated divergent Hox3 copies, named Shox genes, that are required during early embryonic development but do not appear to play homeotic functions (Ferguson et al., 2014; Livraghi, 2017; Mulhair et al., 2023). A lncRNA and two miRNAs were identified in the intergenic region between abd-A and Abd-B in the silkworm (Wang et al., 2017; Wang et al., 2019). In butterfly wings, the regulation of Ubx shows strong patterns of segment-specific regulation at two levels. First, the Ubx transcript has been consistently identified as the most differentially expressed mRNA between the two wing sets (Hanly et al., 2019; Wang et al., 2022). Second, comparison of ATAC-seq signals reveal that forewing vs. hindwing have identical open-chromatin profiles during development across the genome, except at the Ubx gene itself (Lewis et al., 2019; van der Burg et al., 2019). Thus, the ability of the Ubx locus to be robustly activated in hindwings and repressed in forewings is likely driving most subsequent differences between these tissues. In this study, we provide an initial assessment of the regulation of the Ubx locus during butterfly wing development. To do this, we leverage genomic resources and CRISPR mutagenesis with a focus on two laboratory species belonging to the Nymphalinae sub-family, J. coenia and Vanessa cardui (Livraghi et al., 2017; Martin et al., 2020; van der Burg et al., 2020; Mazo-Vargas et al., 2022). We identify putative regulatory regions likely involved in the repression and activation of Ubx expression, and discuss the potential mechanisms restricting it to hindwings. Finally, we describe a collection of spontaneous wing homeotic mutants in Heliconius spp. and elaborate on the categories of mutations that could underlie these phenotypes by misregulating Ubx.
Results
Gene expression at the Ubx locus during wing development
We provide annotations of the Ubx genomic region in four Nymphalinae butterflies (Figure 1A). These feature existing genomic resources for our model species J. coenia and V. cardui (van der Burg et al., 2020; Lohse et al., 2021b; Zhang et al., 2021), as well as for Aglais (Nymphalis) io (Lohse et al., 2021a). The publicly available annotations for these three species include evidence from developmental transcriptomes, and we added to this set a manual annotation of the Ubx locus from the oak leaf butterfly Kallima inachus, for which forewing vs. hindwing transcriptomes have been sequenced across a replicated developmental time series (Yang et al., 2020; Wang et al., 2022).
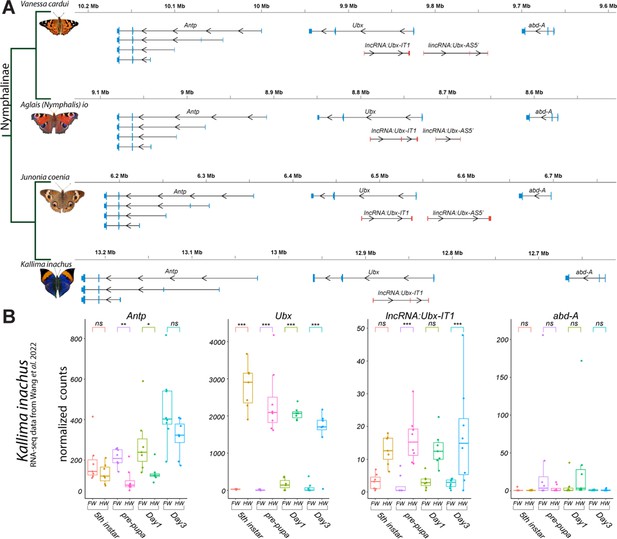
Annotation of the Ubx genomic interval in four butterflies of the Nymphalinae sub-family.
(A) Genomic intervals spanning Antp, Ubx, and abd-A, featuring published transcript annotations from NCBI Reference Genomes of V. cardui and A. io, and manual re-annotations of the J. coenia and K. inachus genomes using published RNAseq dataset (see Methods). Exons are shown with coding (thick) and non-coding (thin) sections. No lincRNA:Ubx-AS5’ transcripts were detected in K. inachus. (B) Expression profiling of transcripts of the Ubx region in K. inachus, based on a reanalysis of published wing RNA-seq transcriptomes (Wang et al., 2022). Expression levels are plotted as DESeq2 normalized counts plots. Pairwise Wald tests adjusted for multiple test correction each assess differential expression between forewings and hindwings. ns: non-significant; *: p<0.05; **: p<0.01; ***: p<0.001.
All Nymphalinae show a similar organization of the region spanning Ubx. Interestingly, the first intron of Ubx encodes a long non-coding RNA in opposite orientation to Ubx, that we dub here lncRNA:Ubx-IT1 (abbr. Ubx-IT1), based on the recommended nomenclature (Seal et al., 2023). Orthologous versions of Ubx-IT1 are detected in most NCBI RefSeq genome annotations throughout Lepidoptera (e.g. the ncRNA NCBI:XR_960726 in Plutella xylostella), implying it is a conserved feature of the Ubx locus in this insect order. Finally, both annotations from V. cardui, A. io, and J. coenia show a long intergenic non-coding transcript starting in antisense orientation about 10–15 kb 5’ of Ubx, that we dub here lincRNA:Ubx-AS5’ (abbr. Ubx-AS5’). This transcript was neither detected in K. inachus transcriptomes nor in RNA datasets outside of the Nymphalinae sub-family, and could be specific to this lineage. Next we reanalyzed the K. inachus wing transcriptomes (Wang et al., 2022), and profiled the expression of Ubx region transcripts during wing development (Figure 1B). As expected from previous studies (Hanly et al., 2019; Paul et al., 2021; Merabet and Carnesecchi, 2022; Wang et al., 2022), Ubx showed a strong expression bias in hindwings, spanning the larval imaginal disks to the intermediate pupal stage. Of note, Ubx is confined to the peripodial membranes of insect T2 wing appendages (Weatherbee et al., 1998; Weatherbee et al., 1999; Prasad et al., 2016), which may explain residual detection in some of the forewing samples here. Ubx-IT1 was significantly enriched in hindwings compared to forewings, albeit at ~100-fold lower count levels than Ubx in the same samples. The Hox gene Antp showed a minor forewing enrichment, confirming that while Ubx expression is robustly repressed in T2 forewing tissues, Antp expression is permitted in both T2 and T3 appendages (Matsuoka and Monteiro, 2021; Matsuoka and Monteiro, 2022; Paul et al., 2021). Expression of abd-A was undetected in most wing samples.
Chromatin 3D conformation reveals a Boundary Element between Antp and Ubx
Genome-wide Hi-C sequencing can be used to generate heatmaps that capture the conformation of 3D chromatin in tissues, and has been used extensively to study Drosophila Hox cluster organisation into TADs that prevent regulatory crosstalk between adjacent genes (Ibragimov et al., 2023; Moniot-Perron et al., 2023). Here, we used Hi-C to assess the 3D chromatin architecture of the Hox cluster interval in the butterfly J. coenia, using existing datasets that were generated from fifth instar larval forewings (van der Burg et al., 2020; Mazo-Vargas et al., 2022). In larval forewings, the Hox chromatin conformation landscape consists of three well-delimited TADs, the first one spanning proboscipedia (pb) to Sex comb reduced (Scr), the second one around Antp, and the third one Ubx, abd-A, and Abd-B (Figures 2 and 3A). A Boundary Element (BE), was robustly called (see Materials and methods) at the region separating the Antp and Ubx TADs, situated in the Ubx last intron. Because TAD boundary prediction has a coarse resolution, we arbitrarily define the candidate BE region as a 15 kb interval centered in the Ubx last intron, and dub it Antp-Ubx_BE. A binding motif analysis identified 4 CTCF binding sites in a 1 kb interval within Antp-Ubx_BE, two of which were found in a tightly linked, convergent orientation (Figure 2—figure supplement 1), which is consistent with TAD insulating role in mediating chromatin loop-extrusion (Guo et al., 2015). This concordance between Hi-C profiling and CTCF motif prediction thus suggests that Antp-Ubx_BE region might function as an insulator between regulatory domains of Antp and Ubx.
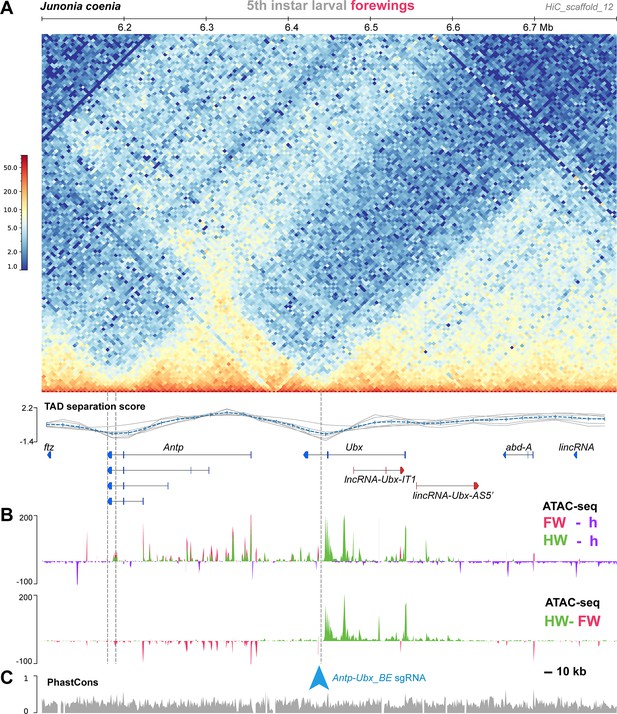
A region of hindwing-specific chromatin-opening is bordered by a TAD BE in the last intron of Ubx.
(A) Hi-C contact heatmap in fifth instar forewings of J. coenia and TAD separation scores around Ubx. A TAD boundary element (Antp-Ubx_BE) is inferred in the last intron of Ubx (vertical dotted line). (B) Differential ATAC-seq profiles, re-analyzed from a previous dataset (Mazo-Vargas et al., 2022). Top: open-chromatin profiles of forewings (FW, magenta), and hindwings (HW, green), respectively subtracted from larval head signal (purple, negative when wing signals at background-level). Bottom: subtractive ATAC-seq profile (HW-FW) revealing hindwing-enriched chromatin in the Ubx locus. Antp-Ubx_BE is in the vicinity of an isolated region of forewing-enriched opening (blue arrowhead). (C) PhastCons genomic alignment scores, with overall alignability suggesting minimal structural variation across this interval in Lepidoptera and Trichoptera.
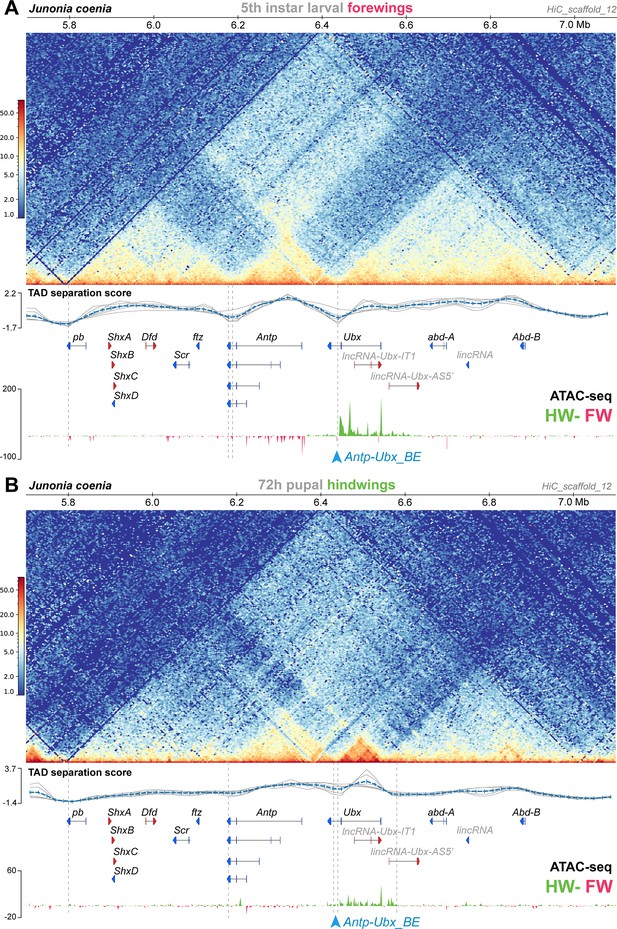
Hindwing-enriched chromatin-opening around Ubx, and the Antp-Ubx_BE boundary, are both maintained in mid-pupal hindwings.
(A) Hi-C heatmap in J. coenia fifth instar larval forewings, and subtractive ATAC-seq profiles at this stage (hindwing-forewing), as expanded from Figure 2 across the Hox cluster. (B) Hi-C heatmap in J. coenia mid-pupal hindwings, and subtractive ATAC-seq profiles at this stage (forewing-hindwing). Inferred TAD boundaries are shown as vertical dotted lines. Blue arrowhead: position of the Antp-Ubx_BE sgRNA.
Differential forewing vs. hindwing chromatin-opening across the Antp-Ubx interval
In flies, the Ubx/abd-A section is organized into regulatory domains that display differential activities across the antero-posterior axis, following what has been called the open-for-business model (Maeda and Karch, 2015; Gaunt, 2022). Here we tested if this pattern extends to butterfly species with a contiguous Hox cluster. To do this, we used ATAC-seq datasets from J. coenia forewing (T2), hindwing (T3), and whole-head tissues sampled across fifth instar larval and early pupal stages, similarly to previous studies (van der Burg et al., 2020; Mazo-Vargas et al., 2022; Van Belleghem et al., 2023). These data reveal that chromatin opening along the Antp/Ubx/abd-A interval is partitioned into several regions showing a transition of T2 to T3 activity (Figure 2B). From the anterior to posterior Hox collinear order (i.e. from Antp towards abd-A), chromatin-opening forms a block of forewing-enriched activity close to Antp and its 5’ region, to a block of activity in both forewings and hindwings that stops at the Antp-Ubx_BE. This region is consistent with the fact that Antp is expressed in both wing pairs (Figure 1B). From Antp-Ubx_BE, the interval including Ubx and a large upstream region is strongly enriched for hindwing opening, consistently with previous studies that found it to be the only genomic region showing this pattern (Lewis et al., 2019; van der Burg et al., 2019). Last, the region surrounding abd-A is devoid of differential open-chromatin activity between forewings and hindwings, in accordance with the exclusion of its expression from thoracic segments (Warren et al., 1994; Tong et al., 2014).
Comparison of 3D conformation and open-chromatin profiles between larval forewings and mid-pupal hindwings
The Hi-C dataset analyzed above was prepared from larval forewings, and forewings do not express Ubx (Figure 1B). Next, we repeated our analysis on a Hi-C dataset generated in pupal hindwings instead (van der Burg et al., 2020), that is in a later-stage tissue expressing Ubx. We found two main differences in this contact landscape compared to the larval forewing (Figure 3). First, the TAD spanning from proboscipedia (pb) to fushi-tarazu (ftz) faded in intensity, while in contrast, the TAD around Antp remained strongly organized. Second, Ubx lost its physical association to the abd-A and Abd-B domains, and gained a TAD boundary situated in the Ubx-AS5’ intron. It is difficult to disentangle effects from staging (larval vs. pupal) and tissues (forewing vs. hindwing) in this comparison. Specifically, these differences we observed may be due to chromatin remodeling between stages, as somewhat expected during metamorphosis (Gutierrez-Perez et al., 2019). Alternatively, it is also possible hindwing development requires Ubx to be insulated from the more posterior enhancers. These issues will require further investigation, for instance using profiling of histone marks, with pairwise forewing-hindwing comparison at single stages. Nonetheless the later hindwing sample showed a maintenance of Antp-Ubx separation. First, while Ubx formed a smaller TAD spanning its coding exons 1–2, this region conserved a domain of hindwing-enriched open-chromatin. Second, boundary prediction called two possible, tightly linked TAD limits in the Antp-Ubx_BE region, showing that the last intron of Ubx still acts as an insulating region. In conclusion, our preliminary comparison of Hox 3D conformation indicates that the Antp-Ubx_BE is relatively stable across two stages and wing serial homologs.
Mutagenic perturbation of Antp-Ubx_BE results in forewing homeosis
Next, we reasoned that the forewing-enriched ATAC-seq peak present in the inferred boundary interval (Figure 4A) might mediate the binding of insulator proteins (Savitsky et al., 2016; Stadler et al., 2017), or act as a transcriptional silencer (Segert et al., 2021). Several genomic features support the former hypothesis. First, the only forewing-enriched ATAC-seq peak across a 150 kb region (spanning the Ubx gene and the Antp-Ubx intergenic region), coincides with the midpoint between the two tentative hicFindTADs boundary predictions inferred from HiC data (Figure 2B). Second, during motif scans conducted across that 150 kb region we found eight predicted binding-sites for the Drosophila CCCTC-Binding Factor (CTCF) clustered in a 5 kb region around the differentially accessible region, and none elsewhere in the last Ubx intron (Figure 4A), suggesting the forewing-enriched ATAC-seq peak may function as a transcriptional insulator (Gambetta and Furlong, 2018; Postika et al., 2018; Kyrchanova et al., 2020; Kaushal et al., 2022). Last, the two candidate CTCF binding motifs that are within the forewing-enriched ATAC-seq peak are also conserved across Lepidoptera and Trichoptera (Figure 2—figure supplement 1), two lineages that diverged around 300 Mya (Jusino et al., 2019; Thomas et al., 2020).

CRISPR perturbation of Antp-Ubx_BE results in FW➞HW homeoses.
(A) Antp-Ubx_BE sgRNA targeting (cyan triangle) of a FW-enriched ATAC-peak (magenta) within the Ubx last intron. (B–C) Two examples of J. coenia Antp-Ubx_BE crispants showing mosaic FW➞HW homeoses, shown in dorsal views. CL-WT: contralateral, horizontally flipped images of forewings from the same individuals. WT HW: wild type hindwings from the same individual and mutant forewing side. Both individuals show disruption of their Radial veins (R1-R5 area). The specimen shown in C displays a partial, ectopic eyespot (asterisk). (D–E) Immunofluorescent detection of the UbdA epitope (green) in fifth instar wings disks of Antp-Ubx_BE crispants, revealing ectopic antigenicity in forewings. WT forewings of similar stage, and HW from the same crispant individuals, are shown for comparison as insets. Green autofluorescence was observed in tracheal tissues. Scale bars: B-C = 500 μm; D-E = 100 μm.
To test this hypothesis, we used CRISPR targeted mutagenesis to perturb Antp-Ubx_BE and assess its functionality, and designed a single sgRNA in a conserved sequence within the forewing-enriched ATAC-seq (Figure 2—figure supplement 1). Remarkably, CRISPR mutagenesis of the Antp-Ubx_BE target induced G0 mutants with homeotic transformations of their forewings into hindwings (Figure 4B–C and Figure 4—figure supplement 1), including identity shifts in patterns, venation, and wing shape. It is important to note that none of the resulting crispants showed hindwing effects. Thus, we can reasonably attribute forewing homeotic phenotypes to indel mutations restricted to the intronic region, without disruption of the Ubx transcript, as this would result in hindwing phenotypes (Matsuoka and Monteiro, 2021; Tendolkar et al., 2021).
Homeotic clones are first visible in Antp-Ubx_BE crispants at the pupal stage, with streaks of thinner cuticle, sometimes associated with local necrosis or with suture defects in the ventral midline, in particular where leg and wing pouches adjoin (Figure 4—figure supplement 2). When dissected out of the pupa, these mutant forewings also show streaks of more transparent wing epithelium, concomitantly with the territories of thinner cuticle above, and PCR genotyping of affected pupal wing tissue confirmed the presence of CRISPR-induced mutations at the Antp-Ubx_BE target site (Figure 4—figure supplement 3). Color pattern homeotic clones were salient at the adult stage, with for example, clonal losses of the forewing specific white-band, and partial acquisitions of the large M1-M2 hindwing eyespot. In one specimen, an ectopic, partial M1-M2 hindwing eyespot appeared in the R5-M1 region, suggesting a perturbation of the eyespot induction process in this wing. Nymphalid forewings have five radial veins (R1-5), which provide sturdiness for flight (Wootton, 1993), while hindwings have only two Radial veins. Forewing homeotic mutants showed mosaic venation defects in the Radial vein area (Figure 4B). Finally, higher expressivity mutant forewings were smaller and rounder, reminiscent of hindwing shape.
Next, we dissected fifth instar larval wing disks from developing Antp-Ubx_BE crispants, and monitored the expression of Ubd-A (Ubx and Abd-A epitopes), normally restricted to the hindwing and only present in the forewing peripodial membrane (Weatherbee et al., 1999). Crispants showed forewing clones with strong ectopic expression of Ubd-A (Figure 4D–E and Figure 4—figure supplement 4). This result supports the inference that Antp-Ubx_BE forewing homeoses are due to the de-repression of Ubx in this tissue.
Mutational interrogation of lncRNA-encoding regions at the Ubx locus
We used CRISPR mutagenesis to test the function of the two lncRNA-encoding loci at the Ubx locus. Mutagenesis of the Ubx-IT1 first exon in J. coenia, and of the Ubx-IT1 promoter in V. cardui, both resulted in crispants with small homeotic phenotypes in forewings and hindwings (Figure 5 and Figure 5—figure supplement 1). This result contrasts with Ubx exon mKO experiments, which only generate hindwing phenotypes (Tendolkar et al., 2021). Given the scarcity of Ubx-IT1 crispants obtained (11 out of 236 adults), and the small size of the homeotic clones within them, we infer the occasional phenotypes may be due to rare alleles. Thus, rather than evidence of functionality of the Ubx-IT1 transcript, the homeotic phenotypes may rather reflect the effects of regulatory perturbation on Ubx itself, with some random mutations in this intronic region resulting in hindwing Ubx loss-of-function, and some others triggering derepression in forewings. Likewise, next we mutagenized the first exon of Ubx-AS5’, located upstream of the Ubx promoter, and obtained twelve hindwing mutants and a single forewing mutant (Figure 6 and Figure 6—figure supplement 1). As with Ubx-IT1 CRISPR experiments, these results may be explained by regulatory disruption of Ubx transcription, with a higher ratio of hindwing phenotypes compared to forewings linked to the proximity of the Ubx promoter. Overall, we conclude that the mutational interrogation at these loci can result in dual loss (hindwing) and gain (forewing) of Ubx function effects. Deciphering whether or when these effects affected Ubx expression via local cis-regulatory modules, impairment of lncRNA transcripts, or larger indels overlapping with Ubx exons, will require further study (see Discussion).
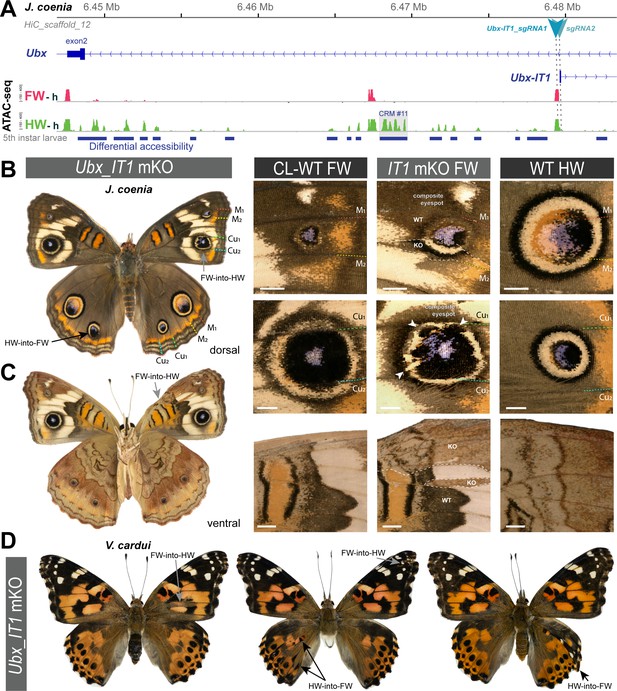
Rare, dual homeoses obtained from CRISPR mutagenesis of the lncRNA_Ubx-IT1 5’ region.
(A) Genomic context of the sgRNA targets (here shown in J. coenia), in the promoter and first exon of the non-coding Ubx-IT1 transcript. (B–C) Dorsal and ventral views of a J. coenia crispant displaying dual homeoses, that is with both FW➞HW (presumably due to Ubx gain-of-expression), and HW➞FW clones (akin to Ubx null mutations). Insets on the right describe forewing mutant clones (IT1 mKO), in apposition to CL-WT (contralateral forewings from the same individual), and WT HW (wild type hindwings from the same individual and mutant forewing side). (D) Examples of dual homeoses obtained when targeting orthologous sites in V. cardui. Scale bars: 1 mm.

Homeoses obtained from CRISPR mutagenesis of the lncRNA Ubx-AS5’ first exon.
(A) CRISPR sgRNA targets (here shown in J. coenia), in the first exon of the non-coding Ubx-AS5’ transcript. (B) A single J. coenia crispant showed a FW➞HW transformation. Insets on the right describe forewing mutant clones (AS5’ mKO), in apposition to CL-WT (contralateral forewings from the same individual), and WT HW (wild-type hindwings from the same individual and mutant forewing side). (C–D) Examples of HW➞FW homeoses obtained in J. coenia or when targeting orthologous sites in V. cardui. Scale bars: 500 μm.
Dual effects of mutagenesis in a putative Ubx cis-regulatory module
In an attempt to probe for Ubx hindwing-specific regulatory sequences, we focused on a~25 kb region in the first intron of Ubx that displays an ATAC-seq signature of hindwing enrichment in open-chromatin relative to forewings, hereafter dubbed CRM11 (Figure 7A). We subdivided this differentially accessible region into four peaks (11 a, b, c, and d). Targeting the ATAC-seq peaks with multiple sgRNAs spanning sub-domains 11 a and 11 c (UbxCRE11a2c5 in V. cardui, 11 a2a3c5c6 in J. coenia), or with a single target in 11 c (UbxCRE11c5 in V. cardui) yielded dual homeoses: FW➞HW and HW➞FW (Figure 7B–D and Figure 7—figure supplement 1). Hindwing effects were reminiscent of Ubx protein coding knockouts (Tendolkar et al., 2021), indicating that these crispant alleles with a hindwing phenotype produce Ubx loss-of-function effects. Individuals with hindwing clones were 2.75 times more common than individuals with forewings in this dataset. Similarly to the lncRNA loci perturbation experiments, dual homeoses may indicate the presence of hindwing activators and forewing repressors in the CRM11 region, with various CRISPR alleles producing a spectrum of indels and effects (see Discussion). It is noteworthy that while single-target experiments showed little lethality (55% hatching rate), dual or quadruple injection mixes resulted in low hatching rates of injected embryos (~10%). Multiple targeting thus appears to induce high-rates of embryonic lethality, possibly due to chromosomal damage (Cullot et al., 2019; Zuccaro et al., 2020). Dual targeting with a2 +c5 also yielded partial HW➞FW homeoses in V. cardui under the form of ectopic white eyespot foci phenotypes (Figure 7E), as occasionally observed in Ubx null crispants (Tendolkar et al., 2021), seemingly due to hypomorphic or heterozygous allelic states.

CRISPR perturbation of Ubx CRM11 generates occasional dual homeotic phenotypes.
(A) Overview of ATAC-seq differential chromatin accessibility profiles (hindwing - head tissues, green; forewing - head tissue, magenta) across the Ubx first exon. Several regions show differential opening between wings, one of which (CRM11), was targeted here for CRISPR perturbation (sites a2 and c5 indicate sgRNA targets). (B) Dual homeosis phenotypes obtained in V. cardui following dual-targeting of UbxCRE11a2c5, including homeoses in color patterns and scale morphology. (D) Additional example of a V. cardui UbxCRE11a2c5 crispant with a forewing phenotype (gain of hindwing hair patches, arrowheads). (E) Example of mild hindwing homeoses showing a white eyespot focus on the dorsal and ventral sides. These effects were previously shown to occur in coding Ubx CRISPR knock-out experiments (Tendolkar et al., 2021). Contralateral (CL) WT wings are shown for comparison with mutant wings (B–E). Colored dashed lines: wing veins. Scale bars: 500 μm.
Next, we focused on a single target shared between both V. cardui and J. coenia in the 11b sub-domain. A whole genome alignment between 23 lepidopteran species and 2 trichopteran species indicated that region 11b is deeply conserved, suggesting important functional constraints on its sequence (Figure 7—figure supplement 2A–B). Mutagenesis of 11b using a single guide RNA (Ubx11b9) yielded a relatively high hatching rate (mean = 51.8 %), indicating a rare occurrence of the deleterious mutational effects observed in multiple targeting (see above). Four J. coenia crispants and two V. cardui crispants were obtained, all exclusively showing hindwing phenotype devoid of forewing effects. HW➞FW homeoses included a variety of phenotypes all seen in Ubx CDS mutants (Tendolkar et al., 2021), including transformations of the orange Discalis elements and the white band in J. coenia, and partial shifts in eyespot identity (Figure 7—figure supplement 2C). Together the consistency in direction of transformations and the deep conservation of the 11b region suggests it may encode an enhancer necessary for the transcriptional activation of Ubx in hindwings.
A sample of spontaneous homeotic mutants in Heliconius butterflies
Homeotic shifts between forewings and hindwings can occur naturally in Lepidoptera, and have been documented as pattern aberrations in museum specimens (Sibatani, 1980; Sibatani, 1983). As a complement to CRISPR-induced homeoses, we document here a rich sample of forewing/hindwing homeotic mutants in the genus Heliconius, systematically collected by L. E. Gilbert between 1987 and 2016 in captive stocks at UT Austin, as well as in the wild. Across these 15 spontaneous mutants, 12 show HW➞FW clones, against 3 specimens with FW➞HW effects (Figure 8, Figure 8—figure supplements 1–2). Mutant clones in this dataset were always posterior to the M2 vein. Only 2 mosaic phenotypes were found on a dorsal side, with the 13 others appearing ventrally. These homeotic mosaics show pattern shifts with complete fore/hindwing conversions of scale types, as seen for instance in the loss of gray wing coupling scales on posterior ventral forewings (Figure 8A–B), or conversely, in their acquisition in posterior hindwings (arrowheads in Figure 8—figure supplements 1–2). Homeoses also include noticeable local changes in wing shape, particularly in hindwings (asterisks in Figure 8—figure supplements 1–2). Taken together, these effects are akin to CRISPR-induced perturbations at the Ubx locus. We speculate that fore/hindwing homeotic aberrations, found in nature and captive stocks, result from mutations at the Ubx locus itself.

Mosaic forewing homeoses in Heliconius butterfly spontaneous mutants.
Wild-type and mutant sides from the same individuals are shown in each panel, with one side digitally flipped to match left-to-right orientation. (A) Heliconius melpomene rosina, ventral view. Wild-caught in the Osa Peninsula (Costa Rica), October 1989. (B) Heliconius cydno galanthus, ventral view (magnified inset in B’). Stock culture from Organisation for Tropical Studies station, La Selva (Costa Rica), June 1990 (C) Heliconius himera, dorsal view (magnified inset in C’). Stock Culture in the butterfly farm Heliconius Butterfly Works in Mindo (Ecuador), March 2008.
Discussion
An intronic region with ATAC-seq hindwing-enrichment regulates Ubx
All CRISPR targets yielded homeotic phenotypes (Figure 9), with two kinds of interference with Ubx expression – forewing gain-of-function effects, and hindwing loss-of-function effects – and indicating the presence of regulatory sequences (broadly defined), that repress or enhance Ubx expression in this region. It is crucial here to highlight the limitations of the method, in order to derive proper insights about the functionality of the regulatory regions we tested. In essence, butterfly CRISPR experiments generate random mutations by non-homologous end joining repair, that are usually deletions (Connahs et al., 2019; Mazo-Vargas et al., 2022; Van Belleghem et al., 2023). Ideally, regulatory CRISPR-induced alleles should require genotyping in a second (G1) generation to be properly matched to a phenotype (Mazo-Vargas et al., 2022). Possibly because of lethal effects, we failed to pass Ubx locus G0 mutations to a G1 generation for genotyping, and were thus limited here to mosaic analysis. As adult wings scales are dead cells, the genetic material building a given color phenotype is lost at this stage, but we circumvented this issue by genotyping a pupal forewing displaying an homeotic phenotype in the more efficient Antp-Ubx_BE perturbation experiment (Figure 4—figure supplement 3). In this case, PCR amplification of a 600 bp fragment followed by Sanger sequencing recovered signatures of indel variants, with mixed chromatograms starting at the targeted sites. But in all other experiments (CRM11, IT1, and AS5’ targets), we did not genotype mutant tissues, as they were only detected in adult stages and generally with small clone sizes. Some of these clones may have been the results of large structural variants, as data from other organisms suggests that Cas9 nuclease targeting can generate larger than expected mutations that evade common genotyping techniques (Shin et al., 2017; Adikusuma et al., 2018; Kosicki et al., 2018; Cullot et al., 2019; Owens et al., 2019). Even under the assumption that such mutations are relatively rare in butterfly embryos, the fact we injected >100 embryos in each experiment makes their occurrence likely (Figure 9), and we are unable to assign a specific genotype to the homeotic effects we obtained in CRM11, IT1 and AS5’ perturbation assays.
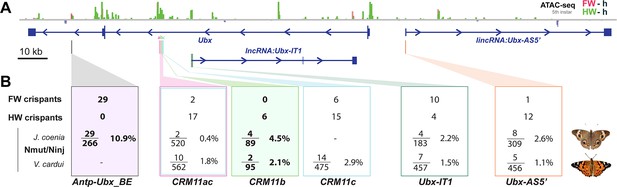
Summary of wing homeosis phenotypes obtained from mutational interrogation.
(A) CRISPR targets at non-coding regions across the Ubx region, here visualized in J. coenia. (B) Summary of injection and adult phenotype data obtained across CRISPR experiments. FW/HW crispants: total number of individuals with forewing or hindwing homeotic clones, regardless of the injected species. Individuals with dual homeosis are counted in both categories. Nmut/Ninj: number of crispants obtained (Nmut), over the number of injected embryos for each species. Bold: experiments with consistent effects in only one segment. See Table 1 for details.
CRISPR mutational interrogation experiments at putative Ubx regulatory regions.
Species | sgRNA(s) | Inj. EmbryosNinj | L1 larvaeNhat | Pupae orL5 larvae | AdultsNadu | CrispantsNmut | Inj. timeh AEL | Cas9:sgRNA ng/µL | Hatching RateNhat/Ninj | Crispant RateNmut/Ninj |
---|---|---|---|---|---|---|---|---|---|---|
J. coenia | Antp-Ubx_BE | 59 | 50 | 50 | 44 | 6 | 2.5–3.5 | 500 : 250 | 84.7% | 10.2% |
118 | 40 | 40 | 31 | 6 | 1.75–2.75 | 250 : 125 | 33.9% | 5.1% | ||
89 | 44 | 44 | 39 * | 17 | 2.25–3.5 | 500 : 250 | 49.4% | 19.1% | ||
Total | 266 | 90 | 134 | 115 | 29 | 33.8% | 10.9% | |||
V. cardui | IT1_sgRNA1 | 204 | 67 | 50 | 50 | 2 | 1–3 | 250 : 125 | 32.8% | 1.0% |
108 | 49 | 31 | 31 | 3 | 2–3 | 125 : 62.5 | 45.4% | 2.8% | ||
145 | 60 | 39 | 39 | 2 | 2.25–3.5 | 500 : 250 | 41.4% | 1.4% | ||
Total | 457 | 176 | 120 | 120 | 7 | 38.5% | 1.5% | |||
J. coenia | IT1_sgRNA2 | 59 | 40 | 7 | 6 | 0 | 0.5–2.5 | 500 : 250 | 67.8% | 0.0% |
124 | 112 | 112 | 110 | 4 | 2.25–4.75 | 500 : 250 | 90.3% | 3.2% | ||
Total | 183 | 152 | 119 | 116 | 4 | 83.1% | 2.2% | |||
V. cardui | AS5_sgRNA1 | 334 | 183 | 57 | 52 | 5 | 2–3 | 250 : 125 | 54.8% | 1.5% |
122 | 87 | 2 | 2 | 0 | 2–4 | 500 : 250 | 71.3% | 0.0% | ||
Total | 456 | 270 | 59 | 54 | 5 | 59.2% | 1.1% | |||
J. coenia | AS5_sgRNA1 | 309 | 181 | 181 | 181 | 8 | 2–4.5 | 500 : 250 | 58.6% | 2.6% |
J. coenia | Ubx11a2+3 +c5+6 | 317 | 18 | - | - | 2 | 1–3 | 500 : 75 ea. | 5.7% | 0.6% |
203 | 35 | 0 | 0 | 0 | 1.5–3.5 | 500 : 75 ea. | 17.2% | 0.0% | ||
Total | 520 | 53 | - | - | 2 | 10.2% | 0.4% | |||
V. cardui | Ubx11a2+c5 | 50 | 5 | 3 | 3 | 2 | 4–4.5 | 500 : 500 | 10.0% | 4.0% |
151 | 29 | 6 | 5 | 2 | 2–2.75 | 500:125:125 | 19.2% | 1.3% | ||
361 | 18 | 13 | 16 | 6 | 0.5–2 | 500:125:125 | 5.0% | 1.7% | ||
Total | 562 | 52 | 22 | 24 | 10 | 9.3% | 1.8% | |||
V. cardui | Ubx11c5 | 168 | 99 | 27 | 26 | 3 | 3.75–4.75 | 250 : 125 | 58.9% | 1.8% |
62 | 22 | 9 | 9 | 2 | 0.5–0.75 | 500 : 250 | 35.5% | 3.2% | ||
131 | 93 | 8 | 8 | 3 | 1.5–3 | 500 : 250 | 71.0% | 2.3% | ||
114 | 63 | 20 | 20 | 6 | 3.5–4.5 | 500 : 250 | 55.3% | 5.3% | ||
Total | 475 | 277 | 64 | 63 | 14 | 58.3% | 2.9% | |||
V. cardui | Ubx11b9 | 32 | 18 | 6 | 5 | 1 | 1.25–2.25 | 500 : 250 | 56.3% | 3.1% |
63 | 49 | 9 | 6 | 1 | 3.5–4.5 | 500 : 250 | 77.8% | 1.6% | ||
Total | 95 | 67 | 15 | 11 | 2 | 70.5% | 2.1% | |||
J. coenia | Ubx11b9 | 41 | 13 | 13 | 13 | 3 | 2.5–4 | 125 : 62.5 | 31.7% | 7.3% |
48 | 21 | 14 | 14 | 1 | 2–3 | 250 : 125 | 43.8% | 2.1% | ||
Total | 89 | 34 | 27 | 27 | 4 | 38.2% | 4.5% | |||
*: upper estimate, includes 16 fifth instars larvae that were dissected for immunostainings, of which 7 were mutants (as evidenced by ectopic UbdA in forewings), and 3 dissected mutant pupae |
When targeting hindwing-enriched ATAC-seq peaks within the first intron of Ubx – from CRM11 to the hindwing-enriched open-chromatin peak that coincides with the first exon of Ubx-IT1 – we obtained a mixture of hindwing and forewing phenotypes. Given the potential heterogeneity of allele sizes in these experiments, it is difficult to conclude robustly about the function of individual targets. Nonetheless, the phenotypic data and in particular the obtention of dual homeoses suggest we disrupted sequences that are necessary to Ubx activation in hindwings, as well as to its repression in forewings. Bifunctional cis-regulatory elements that can switch between enhancer and silencer roles are prevalent in Drosophila (Gisselbrecht et al., 2020; Segert et al., 2021; Pang et al., 2023). The CRM11 and IT1 targets adjoin or overlap with open-chromatin signals in both wing sets (Figures 5A and 7A), providing circumstantial evidence that these regions might serve as bifunctional elements. Similar observations were recently made in mutational interrogation experiments of the butterfly WntA patterning gene (Mazo-Vargas et al., 2022). Alternatively, silencers and enhancers may be tightly linked and interact in close proximity to shape gene expression (Méndez-González et al., 2023), implying in our case that forewing and hindwing phenotypes are mediated by alleles spanning adjacent but distinct elements. A formal test of these mechanisms would require germline transmission and genotyping of these alleles, which was unsuccessful in our attempts at crossing Ubx cis-regulatory crispants. In contrast with the dual effects obtained when targeting CRM11a+c (Figure 9), CRM11b perturbation resulted in hindwing-limited effects, and may suggest that an Ubx enhancer was consistently compromised in this specific dataset. The high lethality and small size of mutant wing streaks suggest that only individuals with sparse, small mutant mitotic clones can survive to the adult stage. If this is true, CRM11 may contain pleiotropic enhancers that are vital for normal Ubx function at earlier stages, but expression-reporter studies will be required to test this.
Parsing lncRNA-encoding regions – correlation or cause?
LncRNAs are emerging as important regulators of gene expression and developmental processes (Zhang et al., 2019; Statello et al., 2021). IT1 targeting generated a majority of forewing phenotypes, suggesting perturbation of Ubx repression in the T2 segment. However, IT1 showed low expression in forewing RNAseq datasets from K. inachus, and higher expression in the hindwing (Figure 1B), a pattern inconsistent with a repressive role of the antisense IT1 transcript on Ubx expression. It is generally challenging to disentangle the effects of transcription of a non-coding element from the potential effects of adjacent enhancers (Natoli and Andrau, 2012; Pease et al., 2013). Therefore, an alternative explanation would be that the phenotypes are confounded by the overlap and proximity to open-chromatin regions, which may play cis-regulatory roles on Ubx via DNA-protein interactions, rather than via the lncRNA. If this is the case, it is possible that the targeted Ubx-IT1 site, which yielded homeoses in both directions and bears both forewing and hindwing open-chromatin, is a bifunctional cis-regulatory element that can shift regulatory activities between these tissues (Gisselbrecht et al., 2020). Targeted mutagenesis of the Ubx-AS5’ first exon mainly generated hindwing phenotypes, although with a relatively low-efficiency. Because this target is about 10 kb away from the Ubx promoter itself, it is plausible that the observed phenotypes were due to large deletions reaching the promoter region of Ubx. As mutational interrogation alone cannot discern if phenotypic effects arose from regulatory failure at the chromatin or transcript level, determining if AS5’ and IT1 are functional lncRNAs will need further examination. Of note, a systematic in-situ hybridization survey (Pease et al., 2013) showed that Drosophila embryos express an antisense transcript in its 5’ region (lncRNA:bxd), as well as within its first intron (lncRNA:PS4). It is thought that Drosophila bxd regulates Ubx, possibly by transcriptional interference or by facilitation of the Fub-1 boundary effect (Petruk et al., 2006; Ibragimov et al., 2023), while the possible regulatory roles of PS4 remain debated (Hermann et al., 2022). While these dipteran non-coding transcripts lack detectable sequence similarity with the lepidopteran IT1 and AS5’ transcripts, further comparative genomics analyses of the Ubx region across the holometabolan insect phylogeny should clarify the extent to which Hox cluster lncRNAs have been conserved or independently evolved.
A TAD boundary element likely acts as an insulator preventing Ubx forewing expression
Tight maintenance of TAD boundaries at the Hox locus is crucial for accurate segment identity and is facilitated by insulator proteins (Stadler et al., 2017; Gambetta and Furlong, 2018; Ramírez et al., 2018). The Antp-Ubx_BE element we targeted is in a good position to block interactions between Antp and Ubx (Figures 2–3). Consistent with this idea, the last intron of Ubx contains 8 CTCF binding motifs that are all clustered within 5 kb around the forewing-enriched ATAC peak, including two sites at highly conserved positions that are only 100 bp away from the CRISPR target (Figure 2—figure supplement 1). CTCF sites prevent cross-talk between regulatory domains in the fly BX-C complex, and result in Hox misexpression when deleted (Postika et al., 2018; Kyrchanova et al., 2020; Kaushal et al., 2022; Kahn et al., 2023). Thus, the density of CTCF sites in this region may be indicative of a bona fide insulator active in forewings. CRISPR mutagenesis of Antp-Ubx_BE generated FW➞HW homeoses associated with a gain of UbdA antigenicity in forewings, with no effects in the other direction, in stark contrast with other targets (Figure 9B). This suggests a possible loss of the TAD boundary in the crispant clones, resulting in a TAD fusion or in a long-range interaction between a T2-specific enhancer and Ubx promoter. Similar deletion alleles resulting in a TAD fusion and misexpression effect have been described at the Notch locus in Drosophila (Arzate-Mejía et al., 2020), in digit-patterning mutants in mice and humans (Lupiáñez et al., 2015; Anania et al., 2022), or at murine and fly Hox loci depleted of CTCF-mediated regulatory blocking (Narendra et al., 2015; Gambetta and Furlong, 2018; Kyrchanova et al., 2020). It will be interesting to profile the binding of insulator proteins and transcriptional repressors across the butterfly Hox TAD landscape to shed more light onto the mechanisms of Ubx insulation, using in vivo assays (Bowman et al., 2014), or in silico predictions that take advantage of updated binding matrices for insect insulator proteins (Mitra et al., 2018). Of note, our CRISPR target is adjacent to an hindwing-enriched peak that also presented CTCF binding sites (Figure 4A). Following a similar logic, this site could be a candidate insulator specific to Ubx-expressing tissues like the hindwing, a hypothesis that will require further testing. Lastly, it is worth noting that the Antp/Ubx TAD boundary we identified is intragenic, within the last intron of Ubx. It is unclear if this feature affects transcription, but this configuration might be analogue to the Notch locus in Drosophila, which includes a functional TAD boundary in an intronic position (Arzate-Mejía et al., 2020).
Making sense of spontaneous wing homeotic mutants
In this article, we documented a large sample of spontaneous homeotic mutants obtained in Heliconius spp. All homeotic clones were limited to the wing posterior compartments (i.e. posterior to the M2 vein), possibly because of parasegmental, compartment-specific regulatory domains that played historic roles in the study of Drosophila BX-C regulation (Maeda and Karch, 2015). Sibatani documented in Lepidoptera that “the mosaics of F/H homeosis tend to occur most frequently in the posterior half of the wing, the boundary of the anterior and posterior halves occurring somewhere in space M1-M2” (Sibatani, 1983). Our collection of spontaneous Heliconius mutants only displayed clones in posterior regions, consistently with this trend. However, our CRISPR perturbation assays of J. coenia and V. cardui cis-regulatory regions also generated anterior clones, with all targets. Deciphering how butterfly Ubx regulation is compartmentized between parasegmental or wing antero-posterior domains will require additional investigation. Most Heliconius homeoses were in the hindwings (i.e. putative Ubx loss-of-expression clones), and among these, all but one were ventral (Figure 4—figure supplements 1–2). Three mutants showed forewing homeoses (i.e. putative Ubx gain-of-expression clones), two of them ventral and one of them dorsal (Figure 8). The systematic reviews of wing homeosis in Lepidoptera found that forewing homeoses are almost as common as hindwing ones (Sibatani, 1980; Sibatani, 1983). Our mutational interrogation assays, while coarse in nature, revealed the existence of activating and repressing cis-regulatory sequences at the Ubx locus itself. Spontaneous FW↔HW homeoses observed in butterflies and moths may thus result from somatic mutations or transposition events at this locus.
Materials and methods
Genome annotations and transcriptomic analysis
Request a detailed protocolNymphalid genome sequences of the Hox cluster and their annotations were extracted from the NCBI Assembly and Lepbase online repositories (Challi et al., 2016; Kitts et al., 2016) as follows: V. cardui from NCBI ilVanCard2.1 and LepBase Vc_v1; A. (Nymphalis) io from NCBI ilAglIoxx1.1; J. coenia from Lepbase Jc_v2; P xylostella from NCBI ilPluXylo3.1. The Ubx regions from ilVanCard2.2, Vc_v1, and Jc_v2 were manually re-annotated using wing transcriptome data on the NCBI SRA (BioProjects PRJNA661999, PRJNA293289, PRJNA237755, PRJNA385867, and PRJNA498283) The genome sequence of K. inachus was obtained from the Dryad repository (Yang et al., 2020). Differential gene expression analysis across the K. inachus Ubx locus were carried out using wing transcriptome data available on the NCBI SRA (BioProject PRJNA698433), following a manual re-annotation of a preliminary gene models provided by Peiwen Yang and Wei Zhang (Wang et al., 2022). All transcripts analyses were performed using the STAR intron-aware aligner and DEseq2 expression analysis package as previously described (Love et al., 2014; Dobin and Gingeras, 2016; Hanly et al., 2019; Hanly et al., 2022). Expression levels were calculated as genome-wide normalized counts and pairwise Wald tests were performed to assess differential expression between forewings and hindwings. Multiple test adjustment was performed using Benjamini and Hochberg correction.
Hi-C and ATAC-seq analyses
Request a detailed protocolHi-C data from J. coenia fifth instar larval forewings and 48–72 hr APF pupal hindwings are available at the NCBI SRA BioProject PRJNA641138 (van der Burg et al., 2020). Triplicated ATAC-seq datasets for larval and pupal wing and head tissues of J. coenia and V. cardui (van der Burg et al., 2019; Mazo-Vargas et al., 2022) are available on the NCBI SRA BioProjects PRJNA497878, PRJNA695303, and PRJNA559165. All the ATAC-seq and Hi-C data were re-analysed on J. coenia and V. cardui Ubx genomic regions as previously described (Mazo-Vargas et al., 2022). Briefly, matrices of interactions were constructed by mapping paired reads against the Junonia coenia genome (Mazo-Vargas et al., 2022) using hicBuildMatrix (Ramírez et al., 2018). Matrices from larvae and pupae were normalized using hicNormalize and corrected with the Knight-Ruiz matrix balancing algorithm. The definitions of topologically associating domains (TADs) can be influenced by various factors such as the choice of software, parameters, sequencing depth, and the presence of experimental noise. To ensure reliability, it is recommended to compare TAD calls with independent datasets, such as histone marks or known factors associated with TAD boundaries. In the absence of these specific datasets, we employed a different combination of parameters in the hicFindTADs tool and compared the resulting TAD calls. HiC matrices at 10 kb and 20 kb bin resolutions were utilized, and TAD insulation scores were evaluated using a false-discovery rate correction for multiple testing, with p-value thresholds of 0.01 and 0.005. Consistent TAD boundary calls with negative TAD separation scores were selected to define domain limits at 10 kb and 20 kb matrix resolutions.
CTCF motif binding predictions
Request a detailed protocolThe program fimo was used to scan for the J. coenia candidate TAD boundary region (HiC_scaffold_12:6430000–6444000) for canonical CTCF binding sites, using the positional weight matrix MA0205.1 deposited in the JASPAR database (Holohan et al., 2007; Cuellar-Partida et al., 2012; Castro-Mondragon et al., 2022).
Genomic conservation analyses
Request a detailed protocolWe generated whole-genome alignments of 25 Lepidoptera and 2 Trichoptera reference species from NCBI Assembly using ProgressiveCactus (Armstrong et al., 2020), and HAL tools (Hickey et al., 2013) for converting the resulting HAL file to the MAF format. We provided a species topology tree of 23 Lepidoptera species to PhyloFit (Hubisz et al., 2011) to fit a multiple sequence alignment on the reference J. coenia Ubx locus, using HKY85 as the substitution model. Using PhastCons (Siepel et al., 2005), we then generated conservation score plots using standard parameters (target-coverage=0.45; expected-length=12; rho = 0.1) stored in BED and WIG file formats.
Butterfly rearing and CRISPR microinjections
Request a detailed protocolJ. coenia and V. cardui colonies were maintained at 25 °C and 60–70% relative humidity in a growth chamber with a 14:10 light:dark photoperiod. Larval rearing on artificial diets, egg collection, and microinjections followed previously described methods (Martin et al., 2020; Tendolkar et al., 2021). Cas9:sgRNA heteroduplexes were prepared as previously described (Martin et al., 2020). Frozen aliquots of Cas9-2xNLS (2.5 μL; 1000 ng/μL) and sgRNA (2.5 μL; 500 ng/μL) were mixed in 2:1 and 4:1:1 mass ratios for single and dual target injections, respectively. CRISPR sgRNA targets are listed in Supplementary file 1.
Genotyping
Request a detailed protocolFor verification in DNA lesions at the intended Antp-Ubx_BE site, a pupal wing fragment harboring visible mutant clones (Figure 4—figure supplement 3B”) or control wild-type tissue were PCR amplified using the diluted protocol of the Phire Animal Tissue Direct PCR Kit (ThermoFisher) and a pair of oligonucleotides (Forward: 5’-ACCGATCGTAAACGTCAACTTAACG-3’; Reverse 5’-TACTGCGGTGGCGAGTGAATG-3’), before purification and Sanger sequencing using the reverse primer.
Antibody stainings
Request a detailed protocolFifth instar wing disks were dissected in ice cold Phosphate Buffer Saline (PBS), fixed for 15–20 min at room temperature in methanol-free formaldehyde diluted to 4% in PBS / 2 mM EGTA (egtazic acid), washed in PBS with 0.1% Triton X-100 (PT), stored in PT with 0.5% Bovine Serum albumin (PT-BSA), incubated overnight at 4 °C in PT-BSA with a 1:5 dilution of the anti-UbdA peptide antibody serum (mouse monoclonal FP6.87, Developmental Studies Hybridoma Bank), and washed in PT. A 1:250 dilution of anti-Mouse IgG antibody coupled to AlexaFluor488 or Rabbit AlexaFluor555 was made in PT-BSA and spun down at 14,000 rcf to remove aggregates, and incubated with wings for 2 h at room temperature, before additional washes, incubation in 50% glycerol-PBS with DAPI (4′,6-diamidino-2-phenylindole) nuclear stain, and incubation and mounting in 60% glycerol-PBS with 2 mM of EDTA (Ethylenediaminetetraacetic acid).
Imaging
Request a detailed protocolFull-mount photographs of J. coenia and V. cardui were taken on a Nikon D5300 digital camera mounted with an AF-S VR MicroNikkor 105 mm f/2.8 G lens, with magnified views taken using a Keyence VHX-5000 digital microscope mounted with VH-Z00T and VH-Z100T lenses. Immunofluorescent stainings were imaged on an Olympus BX53 epifluorescent microscope mounted with UPLFLN 4 x/0.13 and 10 X/0.3 objectives.
Data availability
The current manuscript used previously published data that are refererenced in the Materials and methods section. No genomic data have been generated for this manuscript.
-
NCBI BioProjectID PRJNA661999. Vanessa cardui Genome sequencing and assembly.
-
NCBI BioProjectID PRJNA293289. Vanessa cardui Raw sequence reads.
-
NCBI BioProjectID PRJNA237755. De novo transcriptome analysis profiles gene expression underlying seasonal polyphenism in butterfly wing patterns.
-
NCBI BioProjectID PRJNA385867. A single master regulatory gene optix underlies both color and iridescence in butterflies.
-
NCBI BioProjectID PRJNA498283. Contrasting roles of transcription factors spineless and EcR in the highly dynamic chromatin landscape of butterfly wing metamorphosis (buckeye).
-
NCBI BioProjectID PRJNA698433. The genetics of leaf mimicry in Kallima inachus.
-
NCBI BioProjectID PRJNA641138. Genomic architecture and evolution of a seasonal reaction norm [Hi-C] (buckeye).
-
NCBI BioProjectID PRJNA497878. Contrasting roles of transcription factors spineless and EcR in the highly dynamic chromatin landscape of butterfly wing metamorphosis (buckeye).
-
NCBI BioProjectID PRJNA695303. Chromatin landscape of butterfly developing wings.
-
NCBI BioProjectID PRJNA559165. Genomic architecture and evolution of a seasonal reaction norm [ATAC-seq] (buckeye).
References
-
Characterisation of the role and regulation of Ultrabithorax in sculpting fine-scale leg morphologyFrontiers in Cell and Developmental Biology 11:1119221.https://doi.org/10.3389/fcell.2023.1119221
-
JASPAR 2022: the 9th release of the open-access database of transcription factor binding profilesNucleic Acids Research 50:D165–D173.https://doi.org/10.1093/nar/gkab1113
-
CRISPR-Cas9 genome editing induces megabase-scale chromosomal truncationsNature Communications 10:1136.https://doi.org/10.1038/s41467-019-09006-2
-
Optimizing RNA-Seq mapping with STARMethods in Molecular Biology 1415:245–262.https://doi.org/10.1007/978-1-4939-3572-7_13
-
Hox miRNA regulation within the Drosophila Bithorax complex: Patterning behaviorMechanisms of Development 138 Pt 2:151–159.https://doi.org/10.1016/j.mod.2015.08.006
-
Seeking sense in the hox gene clusterJournal of Developmental Biology 10:48.https://doi.org/10.3390/jdb10040048
-
abd-A regulation by the iab-8 noncoding RNAPLOS Genetics 8:e1002720.https://doi.org/10.1371/journal.pgen.1002720
-
Homeotic genes: Clustering, modularity, and diversityFrontiers in Cell and Developmental Biology 9:718308.https://doi.org/10.3389/fcell.2021.718308
-
Conservation and variation in Hox genes: how insect models pioneered the evo-devo fieldAnnual Review of Entomology 58:161–179.https://doi.org/10.1146/annurev-ento-120811-153601
-
PHAST and RPHAST: phylogenetic analysis with space/time modelsBriefings in Bioinformatics 12:41–51.https://doi.org/10.1093/bib/bbq072
-
Hox genes and the evolution of the arthropod body planEvolution & Development 4:459–499.https://doi.org/10.1046/j.1525-142x.2002.02034.x
-
Essential role of Cp190 in physical and regulatory boundary formationScience Advances 8:eabl8834.https://doi.org/10.1126/sciadv.abl8834
-
Assembly: a resource for assembled genomes at NCBINucleic Acids Research 44:D73–D80.https://doi.org/10.1093/nar/gkv1226
-
Genome-wide regulatory adaptation shapes population-level genomic landscapes in heliconiusMolecular Biology and Evolution 36:159–173.https://doi.org/10.1093/molbev/msy209
-
CRISPR/Cas9 as the Key to unlocking the secrets of butterfly wing pattern development and its evolutionAdvances in Insect Physiology 54:85–115.https://doi.org/10.1016/bs.aiip.2017.11.001
-
The regulation of Hox gene expression during animal developmentDevelopment 140:3951–3963.https://doi.org/10.1242/dev.068346
-
Bringing immersive science to undergraduate laboratory courses using CRISPR gene knockouts in frogs and butterfliesThe Journal of Experimental Biology 223:jeb208793.https://doi.org/10.1242/jeb.208793
-
Functional analysis of Ultrabithorax in the silkworm, Bombyx mori, using RNAiDevelopment Genes and Evolution 219:437–444.https://doi.org/10.1007/s00427-009-0305-9
-
Hox dosage and morphological diversification during development and evolutionIn: Seminars in Cell & Developmental Biology 152–153:70–75.https://doi.org/10.1016/j.semcdb.2022.11.009
-
DIVERSITY in binding, regulation, and evolution revealed from high-throughput ChIPPLOS Computational Biology 14:e1006090.https://doi.org/10.1371/journal.pcbi.1006090
-
Evolution of the insect Hox gene cluster: Comparative analysis across 243 speciesSeminars in Cell & Developmental Biology 152–153:4–15.https://doi.org/10.1016/j.semcdb.2022.11.010
-
Noncoding transcription at enhancers: general principles and functional modelsAnnual Review of Genetics 46:1–19.https://doi.org/10.1146/annurev-genet-110711-155459
-
Pattern induction across a homeotic boundary in the wings of Precis coenia (Hbn.) (Lepidoptera: Nymphalidae)International Journal of Insect Morphology and Embryology 24:243–251.https://doi.org/10.1016/0020-7322(95)00004-N
-
Microhomologies are prevalent at Cas9-induced larger deletionsNucleic Acids Research 47:7402–7417.https://doi.org/10.1093/nar/gkz459
-
Identification of non-coding silencer elements and their regulation of gene expressionNature Reviews. Molecular Cell Biology 24:383–395.https://doi.org/10.1038/s41580-022-00549-9
-
Hox dosage contributes to flight appendage morphology in DrosophilaNature Communications 12:2892.https://doi.org/10.1038/s41467-021-23293-8
-
A standardised nomenclature for long non-coding RNAsIUBMB Life 75:380–389.https://doi.org/10.1002/iub.2663
-
Transcriptional silencers: Driving gene expression with the brakes onTrends in Genetics 37:514–527.https://doi.org/10.1016/j.tig.2021.02.002
-
Analysis of the Tribolium homeotic complex: insights into mechanisms constraining insect Hox clustersDevelopment Genes and Evolution 218:127–139.https://doi.org/10.1007/s00427-008-0213-4
-
Wing homoeosis in Lepidoptera: a surveyDevelopmental Biology 79:1–18.https://doi.org/10.1016/0012-1606(80)90069-x
-
A compilation of data on wing homoeosis in LepidopteraThe Journal of Research on the Lepidoptera 22:1–46.https://doi.org/10.5962/p.266811
-
Lessons on gene regulation learnt from the Drosophila melanogaster bithorax complexThe International Journal of Developmental Biology 64:151–158.https://doi.org/10.1387/ijdb.190167rm
-
Gene regulation by long non-coding RNAs and its biological functionsNature Reviews. Molecular Cell Biology 22:96–118.https://doi.org/10.1038/s41580-020-00315-9
-
Ultrabithorax is a micromanager of hindwing identity in butterflies and mothsFrontiers in Ecology and Evolution 9:643661.https://doi.org/10.3389/fevo.2021.643661
-
A multigene phylogeny and timeline for Trichoptera (Insecta)Systematic Entomology 45:670–686.https://doi.org/10.1111/syen.12422
-
Ultrabithorax and the evolution of insect forewing/hindwing differentiationCurrent Opinion in Insect Science 19:8–15.https://doi.org/10.1016/j.cois.2016.10.007
-
Identification and characterization of a new long noncoding RNA iab-1 in the Hox cluster of silkworm, Bombyx mori identification of iab-1Journal of Cellular Biochemistry 120:17283–17292.https://doi.org/10.1002/jcb.28990
-
Leading edge section and asymmetric twisting in the wings of flying butterflies (Insecta, Papilionoidea)Journal of Experimental Biology 180:105–117.https://doi.org/10.1242/jeb.180.1.105
-
Chromosome-level reference genome assembly and gene editing of the dead-leaf butterfly Kallima inachusMolecular Ecology Resources 20:1080–1092.https://doi.org/10.1111/1755-0998.13185
-
Mechanisms and functions of long non-coding rnas at multiple regulatory levelsInternational Journal of Molecular Sciences 20:5573.https://doi.org/10.3390/ijms20225573
-
High-quality genome assembly and comprehensive transcriptome of the painted lady butterfly vanessa carduiGenome Biology and Evolution 13:evab145.https://doi.org/10.1093/gbe/evab145
-
Homeotic gene expression in the wild-type and a homeotic mutant of the moth Manduca sextaDevelopment Genes and Evolution 209:460–472.https://doi.org/10.1007/s004270050279
Article and author information
Author details
Funding
National Science Foundation (IOS-1656553)
- Arnaud Martin
National Science Foundation (IOS-2110534)
- Arnaud Martin
George Washington University (Wilbur V Harlan Research Fellowship)
- Amruta Tendolkar
National Science Foundation (2109536)
- Anyi Mazo-Vargas
Smithsonian Institution (Biodiversity Genomics Fellowship)
- Joseph J Hanly
The funders had no role in study design, data collection and interpretation, or the decision to submit the work for publication.
Acknowledgements
We thank Ling Sheng Loh and the undergraduate researchers from the Martin Lab for assistance with micro-injections and insect rearing, Rachel Canalichio and the GWU Harlan Greenhouse personnel for growing host plants, Patricia Hernandez for sharing microscopes, and Alex Wild for assistance with Heliconius microphotographs at UT Austin. We wish to acknowledge James Lewis and Bob Reed for stimulating insights on open-chromatin biology and the Hox locus, as well as for generating Hi-C libraries published in previous publications that we re-analyzed here. This work was supported by the NSF awards IOS-1656553 and IOS-2110534 to AM, the Wilbur V Harlan Research Fellowship to AT, the NSF Postdoctoral Research Fellowship in Biology 2109536 to AMV, and the Smithsonian Institution Biodiversity Genomics Fellowship to JJH.
Version history
- Sent for peer review:
- Preprint posted:
- Reviewed Preprint version 1:
- Reviewed Preprint version 2:
- Version of Record published:
Cite all versions
You can cite all versions using the DOI https://doi.org/10.7554/eLife.90846. This DOI represents all versions, and will always resolve to the latest one.
Copyright
© 2023, Tendolkar et al.
This article is distributed under the terms of the Creative Commons Attribution License, which permits unrestricted use and redistribution provided that the original author and source are credited.
Metrics
-
- 1,257
- views
-
- 179
- downloads
-
- 2
- citations
Views, downloads and citations are aggregated across all versions of this paper published by eLife.
Download links
Downloads (link to download the article as PDF)
Open citations (links to open the citations from this article in various online reference manager services)
Cite this article (links to download the citations from this article in formats compatible with various reference manager tools)
Further reading
-
- Evolutionary Biology
- Genetics and Genomics
It is well established that several Homo sapiens populations experienced admixture with extinct human species during their evolutionary history. Sometimes, such a gene flow could have played a role in modulating their capability to cope with a variety of selective pressures, thus resulting in archaic adaptive introgression events. A paradigmatic example of this evolutionary mechanism is offered by the EPAS1 gene, whose most frequent haplotype in Himalayan highlanders was proved to reduce their susceptibility to chronic mountain sickness and to be introduced in the gene pool of their ancestors by admixture with Denisovans. In this study, we aimed at further expanding the investigation of the impact of archaic introgression on more complex adaptive responses to hypobaric hypoxia evolved by populations of Tibetan/Sherpa ancestry, which have been plausibly mediated by soft selective sweeps and/or polygenic adaptations rather than by hard selective sweeps. For this purpose, we used a combination of composite-likelihood and gene network-based methods to detect adaptive loci in introgressed chromosomal segments from Tibetan WGS data and to shortlist those presenting Denisovan-like derived alleles that participate to the same functional pathways and are absent in populations of African ancestry, which are supposed to do not have experienced Denisovan admixture. According to this approach, we identified multiple genes putatively involved in archaic introgression events and that, especially as regards TBC1D1, RASGRF2, PRKAG2, and KRAS, have plausibly contributed to shape the adaptive modulation of angiogenesis and of certain cardiovascular traits in high-altitude Himalayan peoples. These findings provided unprecedented evidence about the complexity of the adaptive phenotype evolved by these human groups to cope with challenges imposed by hypobaric hypoxia, offering new insights into the tangled interplay of genetic determinants that mediates the physiological adjustments crucial for human adaptation to the high-altitude environment.
-
- Ecology
- Evolutionary Biology
Seasonal polyphenism enables organisms to adapt to environmental challenges by increasing phenotypic diversity. Cacopsylla chinensis exhibits remarkable seasonal polyphenism, specifically in the form of summer-form and winter-form, which have distinct morphological phenotypes. Previous research has shown that low temperature and the temperature receptor CcTRPM regulate the transition from summer-form to winter-form in C. chinensis by impacting cuticle content and thickness. However, the underling neuroendocrine regulatory mechanism remains largely unknown. Bursicon, also known as the tanning hormone, is responsible for the hardening and darkening of the insect cuticle. In this study, we report for the first time on the novel function of Bursicon and its receptor in the transition from summer-form to winter-form in C. chinensis. Firstly, we identified CcBurs-α and CcBurs-β as two typical subunits of Bursicon in C. chinensis, which were regulated by low temperature (10 °C) and CcTRPM. Subsequently, CcBurs-α and CcBurs-β formed a heterodimer that mediated the transition from summer-form to winter-form by influencing the cuticle chitin contents and cuticle thickness. Furthermore, we demonstrated that CcBurs-R acts as the Bursicon receptor and plays a critical role in the up-stream signaling of the chitin biosynthesis pathway, regulating the transition from summer-form to winter-form. Finally, we discovered that miR-6012 directly targets CcBurs-R, contributing to the regulation of Bursicon signaling in the seasonal polyphenism of C. chinensis. In summary, these findings reveal the novel function of the neuroendocrine regulatory mechanism underlying seasonal polyphenism and provide critical insights into the insect Bursicon and its receptor.