How scent and nectar influence floral antagonists and mutualists
Abstract
Many plants attract and reward pollinators with floral scents and nectar, respectively, but these traits can also incur fitness costs as they also attract herbivores. This dilemma, common to most flowering plants, could be solved by not producing nectar and/or scent, thereby cheating pollinators. Both nectar and scent are highly variable in native populations of coyote tobacco, Nicotiana attenuata, with some producing no nectar at all, uncorrelated with the tobacco's main floral attractant, benzylacetone. By silencing benzylacetone biosynthesis and nectar production in all combinations by RNAi, we experimentally uncouple these floral rewards/attractrants and measure their costs/benefits in the plant's native habitat and experimental tents. Both scent and nectar increase outcrossing rates for three, separately tested, pollinators and both traits increase oviposition by a hawkmoth herbivore, with nectar being more influential than scent. These results underscore that it makes little sense to study floral traits as if they only mediated pollination services.
https://doi.org/10.7554/eLife.07641.001eLife digest
Flowering plants have evolved a number of different approaches to reproduction. Some use their own pollen and self-fertilize, while others use pollen from other nearby plants. This fertilization by other plants is called ‘outcrossing’ and introduces new genetic variation into each generation, which is extremely important for the evolutionary process.
Some flowering plants rely on animals to help with pollination, attracting visitors with floral scents and rewarding the visitors with sugar-rich nectar. But scent and nectar also attract herbivores that damage the plants. This causes a dilemma for flowering plants, which has led some to evolve to not produce scent or to offer no nectar while masquerading as a plant that does. Previous studies into the costs and benefits of such strategies have looked at the effects of either floral scent or nectar, but no-one has uncoupled the effects of these two traits on both pollination and herbivore attack.
Kessler et al. have addressed this issue in wild tobacco plants, which can both self-fertilize and outcross, and which produce varying amounts of scent and nectar. The experiments were conducted under mesh tents and in field trials in the plant's natural habitat: the Great Basin Desert in Utah. Kessler et al. used a gene-silencing technique called ‘RNA interference’ to inhibit the production of scent or nectar, either separately or together. When grown in field trials, under conditions that prevent self-fertilization, these tobacco plants are frequently visited by a hummingbird and three species of hawkmoth. All four of these animals pollinate the tobacco plants, but one of the moths also lays eggs that hatch into caterpillars, which damage the plant. Kessler et al. monitored the effects that the loss of scent, nectar or both had on visits by each pollinator and on outcrossing.
These experiments revealed that scent is essential to attract one hawkmoth species but not for another (called Hyles lineata). Furthermore, while, the hummingbird needs nectar, the H. lineata moth does not; but this moth won't visit flowers that lack both scent and nectar. The experiments also show that, for the moth that lays its eggs on the tobacco plants, both scent and nectar increase pollination and egg laying, but nectar has a stronger effect. Thus reducing nectar, as this tobacco plant does in the wild, is one strategy that can be used to reduce herbivore attack by caterpillars. Together, these findings highlight that it is important to study both herbivores and pollinators when attempting to understand the evolution of floral traits.
https://doi.org/10.7554/eLife.07641.002Introduction
Plants attract pollinators to move pollen from one flower to another to ensure outcrossing and frequently pay for these pollination services by rewarding floral visitors with nectar (Kevan and Baker, 1983; Schemske and Bradshaw, 1999; Raguso and Willis, 2005). Even though it is clear that both floral scent and nectar provide fitness benefits for plants, rewardless flowers have evolved in all major groups of angiosperms (Renner, 2006). Orchids, in particular, have frequently evolved deceptive pollination systems, in which flowers attract pollinators by mimicking mating partners or oviposition sites without offering rewards (Schiestl, 2005). But rewardless, nectar-free, flowers are commonly found within species that normally provide nectar, and this is surprising, as the occasional nectar-free flowers would have a disadvantage if visitors have the sensory abilities to avoid rewardless flowers (Karban, 2015). Early on, theorists (Bell, 1986) recognized that most flowers hide their rewards, for example, deep in the corolla tube, which thwarts the easy visual evaluation of a flower's standing nectar volume and developed an ESS model for the proportion of cheating flowers and discriminating visitors that would be evolutionarily stable. Researchers have since uncovered evidence consistent with the predictions of the model. Gilbert et al. (1991) found that nectar secretion was highly variable within plants of a population and suggested that floral visitors could distinguish between low and high nectar secreting plants. Recent research suggests that hawkmoth pollinators can use humidity as a proxy for the presence of nectar (von Arx et al., 2012).
To examine the importance of nectar for pollination services and to study the fitness advantages of nectar-cheating plants, researchers have used native varieties with reduced nectar accumulation, introgression lines (Brandenburg et al., 2012a, 2012b), artificial flowers (e.g., Ishii et al., 2008), or conducted direct manipulations of nectar quantities by adding artificial nectar to flowers (e.g., Mitchell and Waser, 1992; Jersáková et al., 2008). Ishii et al. (2008) found that pollinators avoided inflorescences with greater numbers of empty flowers. Smithson (2002) added nectar to rewardless orchids, and while the addition changed bee behavior, it did not influence plant fitness. Brandenburg et al. (2012a) found that nectar-deficient Petunia lines produced fewer seeds than did nectar-replete control plants, because Manduca sexta moths reduced their probing times in low-nectar plants, which in turn, reduced pollen transfer and thus seed set.
While rewards keep pollinators moving pollen from one plant to another, other cues, such as floral scent, provide honest signals that advertise the occurrence of the rewards (Wright and Schiestl, 2009). Floral scent is known to play a central role in attracting insect pollinators to flowers (Galen and Newport, 1988; Jürgens et al., 2002; Klahre et al., 2011; Byers et al., 2014; Riffell et al., 2014). The effect of floral scent on the pollination success of single pollinator species has been studied mainly with scent augmentations and additions to existing scent bouquets (e.g., Majetic et al., 2009; Shuttleworth and Johnson, 2010). Shuttleworth and Johnson (2010), for example, showed that single sulphur compounds are responsible for the shift between wasp and fly pollination in Eucomis (Hyacinthaceae). Byers et al. (2014) found altered bumblebee visitation rates in response to single volatile compounds which were added to the scent bouquet of Mimulus species. In most studies, only one pollinator species was investigated at a time, frequently in very specialized model systems, often the sexually deceptive pollination systems of orchids (Schiestl, 2005; Schiestl and Schlüter, 2009). Several studies investigated fitness outcomes of these manipulations. Majetic et al. (2009) for example, found a positive effect on both pollinator visitation and seed production in Hesperis matronalis by augmenting inflorescences with scent extracts. Kessler et al. (2008) genetically manipulated the biosynthesis of the most abundant floral volatile, benzylacetone (BA), in the flowers of the wild tobacco (Nicotiana attenuata) to demonstrate that BA emission is required to maximize both maternal and paternal fitness in the field when the entire native community of floral visitors had access to the flowers. Few studies have investigated the influence of scent and nectar on the entire pollinating community simultaneously.
Floral traits are shaped not only by interactions with mutualists, but also with antagonist, such as herbivores, florivores, seed feeders, or nectar robbers (Strauss and Whittall, 2006; Andrews et al., 2007; Kessler et al., 2013). Both floral scent and nectar are known to influence antagonists and again manipulative experiments have been essential in illuminating these interactions. Floral scents allow florivores to locate their host plants (Theis, 2006; Theis and Adler, 2012). Several studies have shown that individual compounds in floral scent bouquets specifically deter florivores and nectar robbers (Galen et al., 2011; Junker et al., 2011; Kessler et al., 2013) and thus complex floral scents can both attract pollinators and deter antagonists. Nectar quantity has also been shown to influence herbivory, as inferred by adding nectar or sugar solutions to flowers to increase the standing volume of nectar. In both Datura wrightii (Adler and Bronstein, 2004), as well as N. attenuata (Kessler, 2012) nectar addition increased oviposition by M. sexta, a pollinator as an adult as well as a devastating herbivore as a caterpillar for both plant species. The inference from both studies is that plants could reduce their herbivore load by producing only small amounts or no nectar at all.
As suggested by the early ESS modeling efforts (Bell, 1986), a few nectar-free plants dispersed in a large population of nectar-producing plants could realize a fitness benefit from reduced herbivore loads, if at low frequencies pollinators cannot learn their locations (Gumbert and Kunze, 2001) or otherwise identify their lack of rewards (Smithson and MacNair, 1997; Smithson, 2009). A few studies investigated the influence of floral traits for both pollinators and herbivores at the same time. Theis et al. (2014), for example, found that floral sesquiterpenoids from Cucurbitaceae were the best predictors of flower preference for both the specialist pollinator squash bees and the specialist herbivore cucumber beetle. Schiestl et al. (2014) showed that the herbivory-induced reduction of floral volatiles reduced the attractiveness of flowers to pollinators. These studies however were focusing on floral signals and did not consider the function of floral rewards, and so while the ESS explanation for rewardless flowers being maintained by frequency dependent selection is well established (Bell, 1986), we still lack studies that evaluate the relative selective pressures of mutualistic and antagonistic floral visitors on floral reward provisioning strategies.
In this study, we rigorously investigate the consequences of reducing floral scent and nectar independently and simultaneously by RNAi not only for mutualistic interactions but also for oviposition rate, which is an excellent predictor of future herbivory. We measured outcrossing rates afforded by the entire natural pollinator community in N. attenuata's native habitat, by three specific pollinators (hummingbirds and two hawkmoth species), and oviposition by M. sexta. Different approaches were used in the field and laboratory to study pollination and herbivory. At our field site at the Lytle Ranch Preserve in SW Utah, we conducted common garden experiments using plants silenced by RNAi in the production of floral scent (CHAL; Kessler et al., 2008), floral nectar (SWEET9; Lin et al., 2014), or both (CHALxSWEET9), in comparison to empty vector-transformed control plants (EV). The RNAi constructs silenced only the targeted pathways and were otherwise isogenic, and the transformed plants were morphologically indistinguishable from EV transformed and wild-type (WT) plants. This approach allowed for the study of the entire panoply of interactions simultaneously, including different floral visitors, and herbivores to gain an unbiased picture of the interactions of individual players in the complex web of interactions that occur when flowers advertise for pollinator services.
Results and discussion
To examine the natural genetic variability in floral advertisement and rewards for pollinator services in native populations of N. attenuata plants, seeds from plants from 13 native populations, collected between 1993 and 2009 within a 200 km radius of our field station in the SW USA (Utah, Arizona), were used. The variance in standing nectar volume was assessed by selecting 52 plants in the glasshouse. The average standing nectar volume was 3.3 μL and varied between single genotypes between 1.3 μL and 5.7 μL per flower (Figure 1). In addition to this fourfold difference in nectar volume, we also assessed the variability of BA emission from individual flowers from the same sample of plant populations. The average emission was 5.0 ng BA per flower per night, varying between a minimum emission of 0.5 ng and a maximum of 33.9 ng, equivalent to a 70-fold difference in emission rates (Figure 1). Nectar sugar concentrations were in contrast, rather constant. Sugar concentration in newly opened flowers varied between 14.6 and 22.8%, with an average of 18.2%.
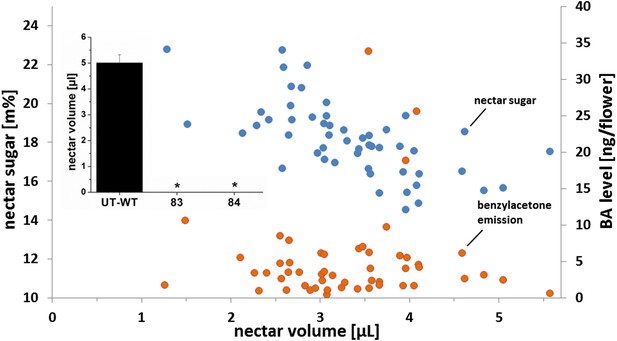
Characterization of 52 plants from different natural populations.
Mean standing nectar volume (n = 6 to 8 flowers/plant), nectar sugar concentration (n = 6 to 8 flowers/plant), and benzylacetone (BA) level (n = 1 flower/plant). Nectar volume and sugar concentration were measured from newly opened flowers at the end of the nectar production period (5–6 am). BA level was measured over the night (8 pm–6 am) and calculated from peak areas normalized using tetralin as an internal standard. Inset: mean (+SE) standing nectar volume in newly opened flowers at the end of the nectar production period (5–6 am) in three native phenotypes (Ut-WT, 83, and 84; n = 7–9) found within a separate sample of 424 native Nicotiana attenuata plants that were screened for the presence of nectar. Asterisks indicate significant differences, as informed by a nonparametric Mann–Whitney U-test p < 0.05.
In a second screening, 424 individual plants were screened for the presence or absence of nectar and scent. Seeds for this experiment were collected from 75 natural populations in Utah, Arizona, and California between 1990 and 2009. Of these, two plants produced no nectar at all. These lines (83 and 84) were inbred for two generations and plants of the T2 generations of both lines also did not produce any nectar in any flowers at all stages of development (Figure 1), demonstrating that the lack of nectar production was heritable. All flowers from all 424 plants in this analysis produced BA in some measureable quantity. We found no evidence for a linear correlation between BA emission and nectar volume (y = 0.1875x + 1.02, R2 = 0.01), BA emission and nectar sugar concentration (y = 0.1233x + 3.89, R2 = 0.01), or nectar volume and nectar sugar (y = −1.4471x + 22.99, R2 = 0.41). While we cannot exclude that genotypes completely lacking floral scent occur in native populations, our results demonstrate that native populations harbor nectar-free ‘cheating’ plants at low frequencies. BA emission however does not occur over the entire night, but is commonly released in a sharp peak in the first part of the night (Kessler et al., 2010), depending on the genotype and the environment. Hence, there are times in the night were one plant is emitting BA, while a neighboring plant is not in genetically diverse populations. Since nectar and scent production were uncorrelated in natural populations, we used RNAi to completely uncouple nectar and scent production in all combinations in an isogenetic background to rigorously examine their costs and benefits.
To investigate the consequences of a lack in nectar, floral scent, and both on pollination and herbivory, EV, SWEET9, CHAL, and CHALxSWEET9 plants were randomly planted 4 m apart in a field plot in the SW USA (Utah) in the plant's native habitat (Figure 2A). Since N. attenuata is a self-compatible plant, anthers were removed from five flowers per plant, and all additional flowers were removed. Hence, seeds of treated flowers were produced entirely from pollinator-mediated outcrossing with neighboring WT plants, which were planted between the rows of transformed plants and were allowed to flower normally. To evaluate the overall pollinator attractiveness of the lines, we allowed floral visitors free access to treatment plants for the flowers' lifetime (3 days). All three lines lacking either floral scent, nectar or both, produced significantly fewer seeds in comparison to similarly antherectomized EV plants (Figure 2B; Friedman signed rank test χ2 = 10.22, df = 3, n = 16–20, p = 0.017). CHAL plants produced only 22.9% (p < 0.001), Sweet9 9.7% (p < 0.001), and CHALxSWEET9 11.6% (p < 0.001) of the seeds produced by EV from outcrossed pollen. The most frequent floral visitors of N. attenuata at night in the field at the time of the experiment were M. sexta, Manduca quinquemaculata, and Hyles lineata hawkmoths (Sphingidae), and Archilochus alexandri hummingbirds (Trochilidae) during the day time. In order to evaluate each species individually, we covered experimental plants at night with mesh cones (Kessler et al., 2010) to only allow hummingbirds to access the flowers. While CHAL (75.6%; Friedman signed rank test χ2 = 7.66, df = 2, n = 24, p = 0.02; p = 0.835) plants produced almost as many seeds as EV plant, SWEET9 plants produced considerably fewer seeds than did EV plants (13.1%; p = 0.074) and significantly fewer seeds than did CHAL plants (17.4%; p = 0.005; Figure 2C).
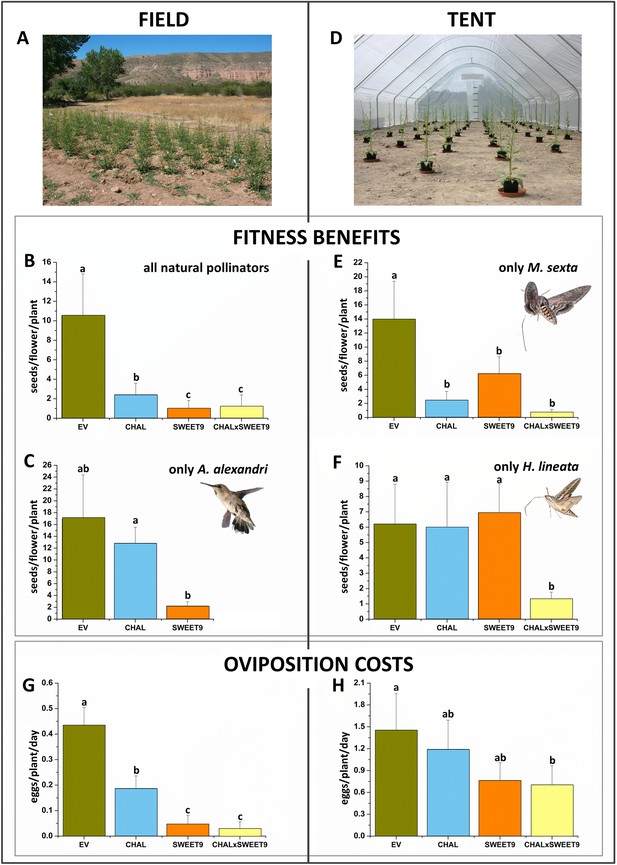
Benefits and cost of floral scent and nectar, as revealed from pollination and oviposition experiments in the field and tent.
Means +SEM of either seed production of antherectomized flowers or oviposition by Manduca sexta on transformed plants silenced in the production of floral scent (CHAL), floral nectar (SWEET9), or both (CHALxSWEET9) in comparison to empty vector control plants (EV). (A) Field plot in N. attenuata's native habitat at the Lytle ranch preserve in Santa Clara, Utah, USA. (B) Seeds sired from pollen transferred by the native community of floral visitors over the complete life span of a flower (3 days). (C) Exclusive pollination by Archilochus alexandri hummingbirds in the field during a 12 hr day. (D) Tent set-up used for single species pollinations in Isserstedt, Germany. Seeds sired from pollination of single M. sexta (E) or Hyles lineata (F) individuals during a 9-hr night. Eggs oviposited per transformed line (n = 10) on different days by the native community of M. sexta in the field (G) or by single individuals in the tent (H). Letters indicate significant differences inferred by a Friedman signed rank test p < 0.05.
Of the three hawkmoth species occurring in the field, we examined two (M. sexta and H. lineata) in a tent set-up. To simulate field conditions for the moths, we used a mesh tent large enough for natural moth pollination behavior (24 m × 8 m; Figure 2D) and examined the consequences of pollination services from single hawkmoths of a given species at a time, something that was not possible to do in the field. To avoid inbreeding or artificial larval diet effects, only moths that had been reared from field-collected eggs and maintained on a plant diet were used.
We used a different moth on each experimental day. M. sexta strongly distinguished between EV and all other lines (Figure 2E; Friedman signed rank test χ2 = 29.90, df = 3, n = 30, p < 0.001). Although SWEET9 lines produced only 44.6% of the seeds produced by EV plants (p = 0.006), the avoidance of plants which produce no floral scent had an even stronger effect on seed set. CHAL plants produced only 17.4% of the seeds of EV (p < 0.001), just as CHALxSWEET9 plants which produced only 5.2% of the seeds produced by EV plants (p < 0.001). Interestingly, H. lineata, a second naturally occurring hawkmoth species, showed a different response to that of M. sexta (Figure 2F; Friedman signed rank test χ2 = 12.35, df = 3, n = 35, p = 0.006). Both CHAL (96.6%; p = 0.853) and SWEET9 (111.69%; p = 0.578) plants produced a number of seeds equivalent to those of EV plants. Only when the flowers lacked both floral traits (CHALxSWEET9) was seed set mediated by H. lineata pollinator activity significantly reduced (21.3%; p = 0.003).
Our results highlight the value of using both floral scent attraction as well as a reward in order to cope with an unpredictable community of pollinators. While floral scent is an essential floral cue for M. sexta, it is clearly not for H. lineata. While nectar is essential for pollination by A. alexandri, the lack of nectar does not reduce the pollination services provided by H. lineata visitations. Though more generalist pollinators such as H. lineata seem to pollinate even when scent or nectar is missing, they strongly reject plants which are unable to produce both floral traits. However, for the more specialized pollinator, M. sexta, both floral scent attraction and nectar reward are important to maximize outcrossing. Although all lines lacking a certain floral trait showed reduced seed set, plants unable to produce nectar still produced more than twice as many seeds than plants unable to produce floral scent. Again, we found a stronger negative effect in plants unable to produce both traits. In contrast, A. alexandri, the only daytime visitor, rejected plants which did not produce nectar, but surprisingly was also less attracted to plants unable to produce floral scent, although visiting flowers at times when BA is not emitted. One explanation for this could be the accumulation of floral scent in nectar (Raguso, 2004), which could influence the taste of the nectar for these pollinators (Kessler and Baldwin, 2007). Another hypothesis could be that hummingbirds also use olfaction and are able to use nectar scent to locate a proper nectar source. Birds, long suspected to use scent in homing and navigation (Keeton, 1974) have recently been suggested to use scent for prey localization (Mantyla et al., 2008).
Hummingbirds, in contrast to hawkmoths, have smaller territories and pollinate flowers only in a small area close to their nests (Norton et al., 1982). This allows them to learn cues and plant positions which are correlated with the presence of food sources (Ackerman et al., 1994; Campbell et al., 1997; Hurly and Healy, 2002) or even with the quality of the food (Pérez et al., 2011). In contrast to hummingbirds, large hawkmoths like M. sexta cover much larger distances each night and rely more on direct perception rather than memory in visiting their food sources (Raguso and Willis, 2003, 2005), as they most likely never visit the same plant twice in nature, although moths are also known to learn when exploring new food sources (Riffell et al., 2013). The inferences we can draw about learning in moths from our results are limited. The pollination assays were conducted with naive moths that lacked experience with rewardless and scentless N. attenuata plants. Experienced H. lineata might have behaved differently, as has been shown in orchid pollinating bumblebees which were able to learn to avoid deceptive orchids (Internicola and Harder, 2012). Our experiments did not directly measure the behavior of the floral visitors and data on the variance in seed set resulting from pollinator visitations, limits the inferences we can draw about pollinator behavior. While there was no evidence that pollinators can sense the presence of nectar, we cannot reject this hypothesis (von Arx et al., 2012).
For future research, it would be interesting to investigate how geitonogamy (Fisogni et al., 2011) would be influenced in rewardless plants. We measured only the pollen flow from one plant to another and not within a given inflorescence. The behaviors of the three tested pollinator species appear to be quite different, and these differences have the potential to influence pollen transfer within an inflorescence and thereby decrease the chance of receiving outcrossed pollen. H. lineata, M. sexta, and M. quinquemaculata, for example, show different patterns of movement in both the field and the tent. While H. lineata moves sequentially from one flower to the next—visiting all flowers within one inflorescence (even visiting the same flower multiple times)—and from one plant to the next plant, both Manduca species visit only a few flowers within an inflorescence before moving on and skipping as many as 10 intervening plants (personal observations).
N. attenuata is capable of producing the full set of seeds of comparable mass and number from self-pollination as from outcrossing (Baldwin et al., 1997). Hence, the plant can compensate for the lack of pollinators by selfing, and the function of producing floral nectar and scent is to ensure outcrossing to maintain genetic variability. Given that N. attenuata seeds can spend hundreds of years in the seed bank before germinating (Preston and Baldwin, 1999), outcrossing will likely be associated with longevity in the seedbank. In other systems outcrossing is thought to increase herbivore resistance in future generations (Bello-Bedoy and Núnez-Farfán, 2011).
How does the production of floral scent or nectar influence M. sexta herbivory? To answer this question, the number of flowers was reduced to the same number on all plants on a given day and oviposited eggs were counted the following morning. In the field, all lines lacking a certain floral trait had reduced loads of eggs (Figure 2G; Friedman signed rank test χ2 = 11.15, df = 3, n = 4, p = 0.0109). On CHAL plants, only 43.1% (p = 0.046) of the eggs found on EV plants were oviposited. The effect was even stronger in plants unable to produce nectar (SWEET9, 10.8%; CHALxSWEET9, 6.5%, p = 0.046). Comparable results were obtained in a similar experiment, conducted in the tent with single moths, (Figure 2H; χ2 = 13.73, df = 3, n = 11, p = 0.0033). CHAL plants received only 81.9% (p = 0.096) of the eggs of EV plants, while SWEET9 received 52.5% (p = 0.058) and CHALxSWEET9 received 48.4% (p = 0.007) of eggs compared with EV. To control for any other leaf-based influences on oviposition, we conducted control experiments with plants without flowers, by using non-flowering plants or plants from which flowers had been removed before the experiment. In the non-flowering stage, no differences in oviposition were found between the different lines (Friedman signed rank test χ2 = 2.81, df = 3, n = 6, p = 0.4214). In the field, oviposition rates (over eight experimental days) were too low for statistical analyses, when plants did not flower. Although, plants unable to produce floral scent had a significantly lower chance of receiving an egg from M. sexta, nectar seems to play a larger role in host choice. In contrast to the pollination results, we found no elevated effect in the CHALxSWEET9 cross compared to the single silenced line, which is consistent with the strong effect of nectar alone. The fact that plants lacking floral scent have reduced ovipositions as well as reduced seed set is probably due to the difficulties hawkmoths have in locating scentless N. attenuata flowers (Kessler et al., 2008).
It is intriguing that it is the reward more than the attractant which influences the female hawkmoth's oviposition decisions, while the attractant plays a similar role as the reward when the same species is acting as a pollinator. Of course our conclusions are context dependent, depending on the frequency of low-nectar or low-scented plants in a native population. Clearly, there needs to be an attractive component (e.g., floral scent) coupled with the nectar reward to allow moths to associate food sources with certain floral signals (Daly and Smith, 2000) or oviposition sites. Similarly, moths would learn to avoid floral advertisements if they are not associated with a reward (Haber, 1984; Smithson, 2001). In an evolutionary context, our findings suggest that for an interaction with M. sexta, N. attenuata plants should minimize their nectar accumulation while maximizing their floral scent emission in order to maximize fitness. Indeed N. attenuata produces tiny amounts of nectar in comparison to other sympatric plant species that compete for M. sexta's pollination services (Raguso et al., 2003; Riffell et al., 2008), and thus seems to have optimized the attractant–reward proportions to maximize its fitness when dealing with this pollinator which is also an herbivore, albeit at a different life stage. D. wrightii, for example, a plant which shares M. sexta as both pollinator and herbivore, is a perennial plant that accumulates 20 times more nectar, produces a large amount of foliage that allows it to better compensate for losses to herbivores, and recruits fewer pollinating species than does N. attenuata (Kessler, 2012).
Pollination success of N. attenuata is ensured by recruiting several pollinator species, which may influence the evolution of floral scent and nectar reward. In the absence of M. sexta, species such as hummingbirds which only pollinate plants capable of producing nectar, and probably will also only continue to pollinate if a sufficient quantity of nectar is available, are important pollen vectors. H. lineata also provides adequate pollination services, but without apparent evolutionary pressure on these N. attenuata floral traits, as this species seems to require neither an attractive signal nor a reward, at least if these plants are surrounded by other nectar and scent producing plants. Moreover H. lineata is also not an herbivore on N. attenuata during its larval stage. These two sphingid species show dramatic differences in their responses to both floral traits. Only M. sexta seems to rely on the cues provided by the flower and distinguishes between honest and cheating plants in a mixed N. attenuata population. For H. lineata, either floral scent or nectar is sufficient for the moth to provide full pollination services. In bee pollinated Brassica rapa plants, the honesty of a floral signal—that a certain scent would consistently lead to a reward—plays a key role in the plants attractiveness to pollinators (Knauer and Schiestl, 2015), something which seems not to be the case for N. attenuata and its pollinators, as we found no association between BA emission and nectar volume, or nectar sugar concentration. Our data are consistent with a trade-off between rewarding pollinators and avoiding herbivores in the evolution of floral nectar in N. attenuata, but why is BA emission so variable? Since antagonists also use floral scent to locate host plants, increasing floral scent emissions would not only increased M. sexta pollination but could also incur costs by possibly increasing florivory or nectar robbing. Carpenter bees, for example, tend to rob more N. attenuata flowers on plants that emit BA than from plants that do not (Kessler et al., 2008) and experimentally enhancing BA emissions increased rates of browsing (Baldwin et al., 1997).
In this study, all pollinators visited N. attenuata at different times between dusk and dawn and it is an interesting question how this temporal order of nectar removal influences the pollination services they provide for the plants. A. alexandri visits N. attenuata approximately 2 h before dusk until dusk and again in the morning after dawn for 2 h. H. lineata visits from 1 h before dusk until 1 h after dawn, and finally both Manduca species pollinate and oviposit after dusk until dawn, with periods of enhanced activity depending on moonlight, temperature, and other conditions. We had expected that these different visiting times would blur the differences in the seed set amongst the lines differing in scent and nectar production when plants were exposed to the entire community of floral visitors at the field station, but the results were just the opposite. The differences between EV plants and SWEET9 as well as CHAL plants were maximized when plants were exposed to the entire community of pollinators at once, suggesting that the behavioral responses of each different pollinator to a given floral trait reinforce each other in ways that can only be understood through more detailed behavioral observations. N. attenuata flowers secrete nectar slowly over the night until approximately 4 am (Kessler, 2012), theoretically keeping a low but constant amount of nectar over the entire night, even if floral visitors removed the majority of nectar in the dusk or in the first half of the night. Unfortunately, we do not know if nectar secretion patterns change after flowers have been visited and nectar has been removed, but from nectar measurements, we know that flowers visited by M. sexta (which invariably removes the standing volume of nectar) again contain a residual amount of nectar in the morning. Similar questions remain about the costs associated with the production of nectar. One would expect that nectaring hummingbirds or white-lined sphinx moths in the dusk could influence M. sexta oviposition by removing all the nectar in the 2 h before M. sexta becomes active. Clearly, there remains a large gap between our understanding of how particular flower traits influence the behavior of the flower visitors and the pollination services that they provide.
Our model system appears particular in that pollinators are at the same time herbivores, but this ecological dilemma is not uncommon for many plant species (Irwin, 2010), and the lessons we draw from our study are likely applicable for other plant systems with differently structured interactions with insects. For example, in the Silene latifolia–Hadena bicruris nursery pollination system, male moths provide a fitness benefit for the plant, while interactions with females come with the substantial costs of seed-feeding caterpillars (Labouche and Bernasconi, 2009). The highly specialized Yucca-Tegiticula pollination/seed predation system would be another example. Plants rarely interact with just one partner and usually face multiple selection pressures from mutualists and antagonists at the same time, which may shape the different floral traits of a plant. In most cases, there will be less and more effective pollinators (Conner et al., 1995; Barthelmess et al., 2006; Matsuki et al., 2008; Miller et al., 2014), as well as herbivores which are attracted by floral signals such as scent or nectar (Adler and Bronstein, 2004; Theis, 2006). Even purely mutualistic interactions like those known from bee pollination systems, probably evolved in the context of avoiding herbivory and excluding less efficient pollinators at the same time. Pollinator networks are known to be highly flexible and change frequently over time within a season (Olesen et al., 2008), as well as between years (Olesen et al., 2011) and this flexibility likely explains why plants recruit additional pollinator species rather than specialize if they rely on outcrossing. Most pollinator networks are nested (Pawar, 2014) and this nestedness is thought to stabilize mutualistic networks (Rohr et al., 2014), reducing interspecific competition and enhancing the number of coexisting species (Bastolla et al., 2009), with the end result of increasing the coexistence of several pollinating species on a plant. The high temporal plasticity in species composition coupled with the low variation in network structure properties in pollination networks makes such a scenario likely (Petanidou et al., 2008).
Flowers face a multidimensional challenge. They have to ensure outcrossing by an unpredictable number of pollinating species and individuals, all of which have different preferences and behaviors, and at the same time need to keep herbivores at bay. Here, we demonstrate that nectar-free, pollinator cheating, varieties of N. attenuata occur in nature, and that producing no nectar can substantially reduce ovipositions by M. sexta, but that this comes with a reduction in pollination services. While cheating on the nectar rewards works for one pollinator species, it does not for others. In contrast, cheating with regards to the attractant floral scent has little influence on M. sexta oviposition, but dramatically reduces outcrossing by M. sexta. All three examined pollinators show different responses to nectar and scent and thus the interactions of multiple species are likely responsible for fine-tuning the evolution of both nectar and floral scent, as both H. lineata, as well as A. alexandri are important pollen vectors in the absence of M. sexta. Both traits in combination are required to maximize maternal fitness in nature particularly in ensuring M. sexta visitations, which we hypothesize delivers the greatest diversity of pollen genotypes compared to H. lineata and A. alexandri. Hence, the close association between N. attenuata and M. sexta may result from M. sexta's ability to move pollen over longer distances than the other pollinators, and thus provide superior outcrossing services among N. attenuata populations which are frequently isolated by the large distances that frequently occur between fires, and hence N. attenuata populations. The long time between fires at any particular location may place a premium on outcrossing rates, as heterozygosity may increase herbivore resistance in future generations (Mescher et al., 2009; Bello-Bedoy and Nunez-Farfan, 2011), as well as longevity in the seedbank. We conclude that herbivores, as well as pollinators shape the evolution of floral traits and that it makes little sense to study floral traits as if they only mediate pollination services.
Materials and methods
Plants, growth conditions, and field plantations
View detailed protocolWild-type (WT) N. attenuata plants, obtained from seeds collected from a native population in 1988 at the DI Ranch (Santa Clara, UT) and subsequently inbred for 22 or 30 generations, were transformed with Agrobacterium tumefaciens (strain LBA 4404) (Krügel et al., 2002) containing the construct pRESC5CHAL to silence N. attenuata chalcone synthase (Kessler et al., 2008), or the construct pSOL8SWEET9 to silence N. attenuata sweet9 (Lin et al., 2014). The vector construction and transformation procedures have been previously described (Krügel et al., 2002). For both CHAL and SWEET9, we choose one line which harbored only one single insertion of the construct and which had completely normal growth and the strongest reductions in either floral volatile or nectar production. Both lines have been fully described previously: CHAL A-07-283-5 (Kessler et al., 2008), SWEET9 A-10-198-4 (Lin et al., 2014). To create a hemizygous cross between CHAL and SWEET9, anthers of CHAL flowers of the T2 generation were removed and flowers hand pollinated with pollen of a SWEET9 (T2 generation) plant. The resulting T3 crossed seeds were used in all field and tent experiments together with the T3 generations of CHAL and SWEET9 plants. As a control, we used EV line A-04-266-3 transformed with pSOL3NC (Bubner et al., 2006), which is known to be completely comparable to wild-type plants (Schwachtje et al., 2008).
Seed germination was performed in both glasshouse and field as described by Krügel et al. (2002). For tent or glasshouse experiments, plants were grown individually in 1 (glasshouse) or 2 L pots (tent) in the glasshouse at 26–28°C under 16 h of light supplied by Philips SON-T Agro 400 (Philips, Germany, http://www.lighting.philips.co.uk/) sodium lights until used for experiments in glasshouse or tent. The tent (Amiran, Kenya, www.amirankenya.com) has a dimension of 8 × 24 m and is about 4 m high. With a distance of 2 m among plants, 60 plants can fit into the tent. The sides and the front the tent is covered with mesh, which allows for air exchange. In the tent, plants were positioned 2 m apart from each other in rows, with three different transformed plants in one row, followed by a row of three WT plants, which served as pollen donors. In total, five plants per transgenic line were positioned in the tent per night for pollination experiments or 10 plants per transgenic line were used for oviposition trials.
In the field, seedlings were transferred into previously hydrated 50-mm peat pellets (Jiffy 703, Always Grows, Sandusky, OH, http://www.alwaysgrows.com/) 14 days after germination and were gradually adapted to the environmental conditions of high sun and low relative humidity of the Great Basin Desert habitat over 14 days by keeping the seedlings in the shade. Adapted size-matched seedlings were transplanted into the field plot at the Lytle Ranch Preserve (Santa Clara). Seedlings were watered every other day until roots were established. The four different transformed genotypes were randomly planted in rows with 4 m between each other and with one pair of WT plants between two transformed plants, which served as pollen donors in pollination experiments. Seeds of the transformed N. attenuata lines were imported under US Department of Agriculture Animal and Plant Health Inspection Service (APHIS) notification numbers 07-341-101, 10-349-101m, and 11-350-102m and the field experiments were performed under notification numbers 06-242-03r, 10-349-102r, and 13-350-101r.
To characterize the phenotypes of all transformed lines, nectar accumulation (Figure 2—figure supplement 1), nectar sugar concentration, floral scent emission (Figure 2—figure supplement 2), as well as leaf volatile emissions (Figure 2—figure supplement 3) were measured in the field. While there were no differences in leaf volatile emission and nectar sugar concentration among the four lines, the lack of nectar in Nasweet9-silenced lines (SWEET9 and CHALxSWEET9), as well as the lack of floral BA emission in Nachal1-silenced lines (CHAL and CHALxSWEET9) were confirmed. No phenological differences, such as plant shape, size, or flower size among these four lines, would suggest a lack of fitness benefit for plants producing no nectar or no scent. In the case of the hemizygous cross between CHAL and SWEET9 (CHALxSWEET9), we observed that occasionally plants started to secrete nectar in late developmental stages. This phenomenon is likely caused by methylation events which occur in aging hemizygous lines (Weinhold et al., 2013). In all experiments, we therefore measured nectar production daily and excluded plants which had lost their initial no-nectar phenotype.
Nectar collection
Request a detailed protocolAll flowers were removed daily before opening. On experimental days, flowers were allowed to open in the evening and inflorescences were covered with plastic bags (Plastibrand, Wertheim, Germany) to exclude pollinators and to retard nectar evaporation. Nectar volume was measured with 25 μL glass capillaries (BLAUBRAND, Wertheim, Germany) on the following morning between 5 and 6 am, at the time of the maximal nectar accumulation (Kessler, 2012). To quantify nectar accumulations, the entire corolla was removed with mild pressure from the flower. The entire nectar remains in the corolla tube and can be squeezed from the tube into a glass capillary (Rothe et al., 2013). Nectar in the capillary was quantified with a ruler. To quantify nectar sugar concentration, we used a portable refractometer (Optech, Sliedrecht, the Netherlands) with a range from 0 to 32% and a resolution of 0.2%. Nectar of 4–7 flowers per plant was measured to assess the variability in the different native phenotypes (single plants), and two flowers per plant were measured in 10 plants of each of our transformed lines, as well as the nectar-free natural phenotypes. For statistics, the average nectar volume or nectar sugar concentration of all measured flowers of a plant was used.
Analysis of plant volatiles from leaf and flower headspaces
Request a detailed protocolPlant volatiles were collected on silicone tubing (ST) and analyzed as described in Kallenbach et al. (2014). Briefly, polyethylene terephthalate (PET) containers were used to enclose leaves (300 mL sampling volume) and flowers (30 mL sampling volume) for headspace sampling. Leaf volatiles were collected in the glasshouse overnight using a similar, fully expanded, mature, non-senescent stem leaf from each plant (n = 7, per genotype). Flower volatiles from EV, CHAL, SWEET9, and CHALxSWEET9 (Figure 2—figure supplement 2) were collected on ST as described (Kallenbach et al., 2014) in the field by trapping flowers overnight (8 pm–6 am) on the first night of opening. Flower volatiles from native populations (Figure 1) were collected using Super-Q traps and analyzed as described in Wu et al. (2008). Empty trapping containers distributed among plants were used as background controls. BA levels were calculated using external calibrations.
Volatile analysis was performed on a TD-20 thermal desorption unit (Shimadzu, Germany, www.shimadzu.de) connected to a quadrupole GC-MS-QP2010Ultra (Shimadzu). Individual STs were placed in 89 mm glass thermo desorption (TD) tubes (Supelco, Germany, sigmaaldrich.com) and desorbed under a stream of nitrogen at 60 ml min−1 for 8 min at 200°C. Desorbed volatiles were cryo-focused at −20°C onto a Tenax adsorbent trap in front of the column. After desorption, the Tenax trap was heated to 230°C within 10 s, and analytes were injected with a 1:20 split ratio onto a ZB-Wax-plus column (30 m long, 0.25 mm i.d., 0.25 μm film thickness; Phenomenex, Germany, www.phenomenex.com) with He as the carrier gas at a constant linear velocity of 40 cm s−1. The TD-GC interface was maintained at 230°C. Two different Gas Chromatograph (GC) oven gradients for the profiling of leaf and flower headspaces were used. For leaf samples, the oven was held at 40°C for 5 min, then ramped to 185°C at 5.0°C min−1, and finally to 230°C at 30°C min−1, where it was held for 0.5 min. For analysis of flower samples, the oven was held at 60°C for 1 min, then ramped to 150°C at 30°C min−1, then to 200°C at 10°C min−1, and finally to 230°C at 30°C min−1 and held for 1 min. Electron impact (EI) spectra were recorded at 70 eV in scan mode from 33 to 400 m/z using a scan speed of 2000 Da s−1. The transfer line was maintained at 240°C and the ion source at 220°C.
Data processing and export were performed using the Shimadzu GCMS solutions software (v2.72). Flower volatiles were analyzed against our reference standards library. For leaf volatiles analysis, raw data files were converted to netCDF format and processed using the R (http://www.r-project.org) packages XCMS (Tautenhahn et al., 2008) and CAMERA (http://www.bioconductor.org/biocLite.R) as described (Gaquerel et al., 2010). After removal of contaminants and known artifact peaks, 94 compounds were detected, and the Metaboanalyst software (5, 6) was used to perform principal components analysis (PCA).
Pollination studies
Request a detailed protocolAs N. attenuata is a self-compatible plant, anthers of flowers were removed in the morning between 5 and 7 am from experimental flowers, just before anthesis (Kessler et al., 2008) and 12 hr before flowers open and are accessible for pollinators. Five flowers per plant were antherectomized. All other floral buds which were about to open within the next 2 days were removed. WT plants located between the experimental plants of the different genotypes were allowed to flower and served as pollen donors. This procedure was used in both tent and field.
In a control experiment in the tent, all four lines used in the experiments were equally able to produce seeds from hand-pollinated flowers (one-way ANOVA: F3, 36 = 1.19, p = 0.33; n = 10). EV plants produced on average 233 ± 23 seeds per flower, CHAL 242 ± 22, SWEET9 209 ± 21, and CHALxSWEET9 265 ± 19. We have no data available for the longevity of seeds of the four lines in the seedbank, which would allow predictions about fitness benefits for future generations of these lines. As such data require years to collect, it is beyond the scope of this study.
In a 2011 field experiment, the response of hummingbirds was tested without including the CHALxSWEET9 cross, as these seeds were not available at this time. To exclude night-time pollinators and thus to evaluate only hummingbird pollination, plants were covered with mesh cones (Kessler et al., 2010) between 8 pm and 6 am. By visual observation, it was ensured that only A. alexandri hummingbirds were visiting the flowers during the times when flowers were accessible to visitors. Bees were not present on the field plot at the time of hummingbird experiments on 2 days in 2011. Experiments planned to investigate the pollination services of the entire community of floral visitors in 2012 and 2013 failed due to fungal or bacterial diseases which killed plants before they started to flower. In 2014, we conducted an experiment, in which we allowed the entire community (day- and night-pollinators) of floral visitors to access treated plants on four experimental days. All plants in this experiment were accessible for floral visitors over the entire lifespan of the atherectomized flowers (3 days). All these experiments have not been influenced by nectar robbing from carpenter bees (Xylocopa spp.). On all experimental days, we monitored their presence, and experiments were not conducted if carpenter bees occurred on the plot, which happened in the later parts of the 2011 and 2014 seasons, when pollination experiments had to be stopped.
In the tent, plants were treated similarly to those of the field experiments, and one single male M. sexta or H. lineata was released in the tent to interact with the experimental plants overnight. Only moths raised on N. attenuata or N. tabacum (M. sexta) or Fuchsia sp. (H. lineata) from field-collected eggs were used for experiments in the tent. In the following morning, plants were moved from the tent into the glasshouse until capsules had matured (approximately 14 days). On each experimental day, new plants and a new moth were used. In total seven M. sexta and seven H. lineata moths were tested in these experiments.
Oviposition trials
Request a detailed protocolThe same plants used for pollination experiments were used for M. sexta oviposition in the field. Flower number of all plants was reduced to five newly opening flowers every afternoon so as to standardize the apparency of each plant's inflorescence to the moths. Each morning, eggs were counted and removed from the plants. The collected eggs, as well as eggs collected from D. wrightii plants at this time of the season were used to rear moths for experiments in the tent. All caterpillars which hatched from these eggs proved to be M. sexta, which suggests that M. sexta accounts for all the oviposition data in our experiments, and not M. quinquemaculata, a closely related species which shares host plants with M. sexta in both the larval and the adult stages. Of the nine experimental days, M. sexta moths oviposited on only four of these days. In the tent experiments, unlike in the pollination trials, no WT pollen donor plants were necessary, which allowed for a larger number of experimental plants in the tent at a time. 10 plants per transformed line were randomly arranged in the tent with a distance of 2 m between plants. Plants were exchanged and rearranged daily, and a freshly mated female M. sexta moth was released into the tent on each day.
Statistic procedures
Request a detailed protocolFor all pollination assays, experiments were conducted over several days. In all cases, the day did not influence the line effect significantly as revealed by a one-way ANOVA p > 0.05. Therefore, each plant was used as a replicate and the average seed number produced from the five flowers on a plant was used for statistical analyses. For oviposition data, days were used as replicates and average values of the different lines were compared. Significant differences for all pollination, oviposition, as well as phenotyping data (nectar volume and floral volatile emissions) data were calculated using a Friedman signed rank test which was performed in R2.11.1 (Crawley, 2007; R Development Core Team, 2007). Control hand-pollinations were compared using a Fisher's PLSD post-hoc test following a one-way ANOVA, which were performed using StatView program version 5.0 (SAS Institute Inc., NC, USA; www.statview.com).
References
-
Pollinator and herbivore attraction to Cucurbita floral volatilesJournal of Chemical Ecology 33:1682–1691.https://doi.org/10.1007/s10886-007-9337-7
-
Patterns and consequences of benzyl acetone floral emissions from Nicotiana attenuata plantsJournal of Chemical Ecology 23:2327–2343.https://doi.org/10.1023/B:JOEC.0000006677.56380.cd
-
The evolution of empty flowersJournal of Theoretical Biology 118:253–258.https://doi.org/10.1016/S0022-5193(86)80057-1
-
The effect of inbreeding on defence against multiple enemies in Datura stramoniumJournal of Evolutionary Biology 24:518–530.https://doi.org/10.1111/j.1420-9101.2010.02185.x
-
Three floral volatiles contribute to differential pollinator attraction in monkeyflowers (Mimulus)The Journal of Experimental Biology 217:614–623.https://doi.org/10.1242/jeb.092213
-
Associative olfactory learning in the moth Manduca sextaThe Journal of Experimental Biology 203:2025–2038.
-
Development and validation of a liquid chromatography-electrospray ionization-time-of-flight mass spectrometry method for induced changes in Nicotiana attenuata leaves during simulated herbivoryJournal of Agricultural and Food Chemistry 58:9418–9427.https://doi.org/10.1021/jf1017737
-
Colour similarity to rewarding model plants affects pollination in a food deceptive orchid, Orchis boryiBiological Journal of the Linnean Society 72:419–433.https://doi.org/10.1111/j.1095-8312.2001.tb01328.x
-
Cue learning by rufous hummingbirds (Selasphorus rufus)Journal of Experimental Psychology 28:209–223.https://doi.org/10.1037/0097-7403.28.2.209
-
Bumble-bee learning selects for both early and long flowering in food-deceptive plantsProceedings. Biological Sciences/the Royal Society 279:1538–1543.https://doi.org/10.1098/rspb.2011.1849
-
Evolutionary ecology: when pollinators are also herbivoresCurrent Biology 20:R100–R101.https://doi.org/10.1016/j.cub.2009.12.005
-
Floral odor bouquet loses its ant repellent properties after inhibition of terpene biosynthesisJournal of Chemical Ecology 37:1323–1331.https://doi.org/10.1007/s10886-011-0043-0
-
Flower scent composition in night-flowering Silene species (Caryophyllaceae)Biochemical Systematics and Ecology 30:383–397.https://doi.org/10.1016/S0305-1978(01)00106-5
-
Advances in the study of behaviorAdvances in the study of behavior, London, NY, Academic Press.
-
Context dependency of nectar reward-guided ovipositionEntomologia Experimentalis et Applicata 144:112–122.https://doi.org/10.1111/j.1570-7458.2012.01270.x
-
Changing pollinators as a means of escaping herbivoresCurrent Biology 20:237–242.https://doi.org/10.1016/j.cub.2009.11.071
-
Insects as flower visitors and pollinatorsAnnual Review of Entomology 28:407–453.https://doi.org/10.1146/annurev.en.28.010183.002203
-
Pollination efficiencies of flower-visiting insects as determined by direct genetic analysis of pollen originAmerican Journal of Botany 95:925–930.https://doi.org/10.3732/ajb.0800036
-
Inbreeding in horsenettle influences herbivore resistanceEcological Entomology 34:513–519.https://doi.org/10.1111/j.1365-2311.2009.01097.x
-
Effect of intrusion pressure on territory size in Black-chinned hummingbirds (Archilochus alexandri)The Auk 99:761–764.
-
Memory for location and visual cues in white-eared hummingbirds Hylocharis leucotisCurrent Zoology 57:468–476.
-
Positive and negative signals regulate germination in the post- fire annualNicotiana Attenuata. Ecology 80:481–494.https://doi.org/10.1890/0012-9658(1999)080[0481:PANSRG]2.0.CO;2
-
BookR: a language and environment for statistical computingVienna: R Foundation for Statistical Computing.
-
Trumpet flowers of the Sonoran Desert: floral biology of Peniocereus cacti and sacred DaturaInternational Journal of Plant Sciences 164:877–892.https://doi.org/10.1086/378539
-
Evolution and ecology taking flight: butterflies as model systems. Rocky mountain biological lab symposium seriesEvolution and ecology taking flight: butterflies as model systems. Rocky mountain biological lab symposium series, Chicago, University of Chicago Press.
-
Plant-pollinator interactions: from specialization to generalizationPlant-pollinator interactions: from specialization to generalization, Chicago, University of Chicago Press.
-
Behavioral consequences of innate preferences and olfactory learning in hawkmoth–flower interactionsProceedings of the National Academy of Sciences of USA 105:3404–3409.https://doi.org/10.1073/pnas.0709811105
-
Pollinator preference and the evolution of floral traits in monkeyflowers (Mimulus)Proceedings of the National Academy of Sciences of USA 96:11910–11915.https://doi.org/10.1073/pnas.96.21.11910
-
On the success of a swindle: pollination by deception in orchidsDie Naturwissenschaften 92:255–264.https://doi.org/10.1007/s00114-005-0636-y
-
Floral isolation, specialized pollination, and pollinator behavior in orchidsAnnual Review of Entomology 54:425–446.https://doi.org/10.1146/annurev.ento.54.110807.090603
-
The missing stink: sulphur compounds can mediate a shift between fly and wasp pollination systemsProceedings of the Royal Society B-Biological Sciences 277:2811–2819.https://doi.org/10.1098/rspb.2010.0491
-
Cognitive ecology of pollination: animal behaviour and floral evolutionCognitive ecology of pollination: animal behaviour and floral evolution, Cambridge, Cambridge University Press.
-
A plant's view of cheating in plant–pollinator mutualismsIsrael Journal of Plant Sciences 57:151–163.https://doi.org/10.1560/IJPS.57.3.151
-
Ecology and evolution of flowersEcology and evolution of flowers, Oxford, Oxford University Press.
-
Fragrance of Canada thistle Cirsium arvense attracts both floral herbivores and pollinatorsJournal of Chemical Ecology 32:917–927.https://doi.org/10.1007/s10886-006-9051-x
-
Floral humidity as a reliable sensory cue for profitability assessment by nectar-foraging hawkmothsProceedings of the National Academy of Sciences of USA 109:9471–9476.https://doi.org/10.1073/pnas.1121624109
Article and author information
Author details
Funding
Max-Planck-Gesellschaft (Max Planck Society)
- Ian T Baldwin
- Danny Kessler
- Mario Kallenbach
- Eva Rothe
- Celia Diezel
- Mark Murdoch
European Research Council (ERC) (Advanced Grant no. 293926)
- Ian T Baldwin
- Celia Diezel
- Mario Kallenbach
- Eva Rothe
National Research Foundation of Korea (NRF) (Global Research Lab program (2012055546))
- Ian T Baldwin
The funders had no role in study design, data collection and interpretation, or the decision to submit the work for publication.
Acknowledgements
This work was funded by the European Research Council advanced grant ClockworkGreen (no. 293926) to ITB, the Global Research Lab program (2012055546) from the National Research Foundation of Korea, and by the Max-Planck-Society. We thank Brigham Young University for the use of the Lytle Ranch Preserve field station, USDA-APHIS for constructive regulatory oversight of the GMO releases, Theresa Erler, Meredith C Schuman, and Antje Wissgott for help with the tent experiments, and the Utah field crew 2014 for help with the emasculation of flowers.
Version history
- Received: March 21, 2015
- Accepted: June 30, 2015
- Accepted Manuscript published: July 1, 2015 (version 1)
- Version of Record published: August 11, 2015 (version 2)
Copyright
© 2015, Kessler et al.
This article is distributed under the terms of the Creative Commons Attribution License, which permits unrestricted use and redistribution provided that the original author and source are credited.
Metrics
-
- 5,000
- views
-
- 973
- downloads
-
- 56
- citations
Views, downloads and citations are aggregated across all versions of this paper published by eLife.
Download links
Downloads (link to download the article as PDF)
Open citations (links to open the citations from this article in various online reference manager services)
Cite this article (links to download the citations from this article in formats compatible with various reference manager tools)
Further reading
-
- Ecology
- Plant Biology
Floral scents and nectar attract both pollinators and other animals that may reduce the plant's fitness, and therefore put flowering plants in a challenging situation.
-
- Ecology
- Evolutionary Biology
Seasonal animal dormancy is widely interpreted as a physiological response for surviving energetic challenges during the harshest times of the year (the physiological constraint hypothesis). However, there are other mutually non-exclusive hypotheses to explain the timing of animal dormancy, that is, entry into and emergence from hibernation (i.e. dormancy phenology). Survival advantages of dormancy that have been proposed are reduced risks of predation and competition (the ‘life-history’ hypothesis), but comparative tests across animal species are few. Using the phylogenetic comparative method applied to more than 20 hibernating mammalian species, we found support for both hypotheses as explanations for the phenology of dormancy. In accordance with the life-history hypotheses, sex differences in hibernation emergence and immergence were favored by the sex difference in reproductive effort. In addition, physiological constraint may influence the trade-off between survival and reproduction such that low temperatures and precipitation, as well as smaller body mass, influence sex differences in phenology. We also compiled initial evidence that ectotherm dormancy may be (1) less temperature dependent than previously thought and (2) associated with trade-offs consistent with the life-history hypothesis. Thus, dormancy during non-life-threatening periods that are unfavorable for reproduction may be more widespread than previously thought.