Pupillometry: Consciousness reflected in the eyes
Most of the tasks that our brain orchestrates in our body are performed outside of our conscious awareness. In addition to breathing, maintaining our balance and keeping our heart beating, these tasks include increasing or decreasing the size of our pupils as our environment becomes darker or lighter. This allows us to see in both darkened rooms and on bright sunny days. Although the area of a pupil can change by as much as a factor of ten (Winn et al., 1994), we are not aware of the small movements in the ocular muscles that open and close our pupils.
Recent studies have painted a complex and fascinating picture of what drives these changes in pupil size. Despite representing the lowest level of visual function, these pupil dynamics can reflect sophisticated processes that are closely linked to our everyday experience (including attention, decision making, and even aesthetic experiences; Binda and Murray, 2015; Hartmann and Fischer, 2014). Therefore, measurements of the pupils, also known as pupillometry, may be used as an indicator for cognitive and perceptual states. This method is also objective and non-invasive, and can, therefore be applied in a wide range of clinical contexts. For example, it enables us to communicate with patients suffering from ‘locked-in’ syndrome – a condition characterized by the paralysis of every muscle except the eye (Stoll et al., 2013).
Now, in eLife, Marco Turi, David Burr and Paola Binda – who are based at research institutes in Pisa, Florence and Sydney – report that fluctuations in pupil size may provide insight into clinical disorders (Turi et al., 2018). The researchers used a well-known optical cylinder illusion that consists of two overlapping sets of black and white dots moving in opposite directions on a 2D plane (Figure 1A). Due to the different speeds of the dots, anyone looking at the dots usually sees a rotating 3D cylinder (Andersen and Bradley, 1998; Video 1 in Turi et al., 2018). However, since the resulting illusion makes it difficult to detect which color is at the front (and, thus, the direction of rotation), our visual system selects one interpretation at any given time. As a result, the perceived rotation switches direction every few seconds (Figure 1B). Such approaches, where the physical stimulus remains constant but our subjective perception changes, have been widely used to study the mechanisms underlying visual awareness (Kim and Blake, 2005).
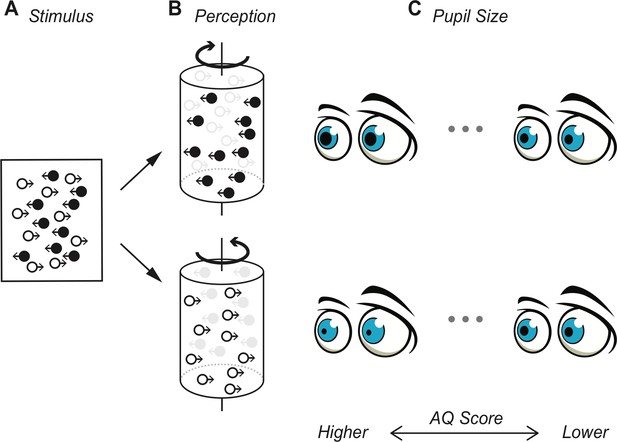
Changes in pupil size depend on subjective visual perception.
(A) Turi et al. used a visual stimulus in which two sets of dots (white and black) moved in opposite directions (thin arrows). (B) To an observer this stimulus appears as a 3D cylinder that rotates in a clockwise direction with the black dots at the front (top), or in a counter-clockwise direction with the white dots at the front (bottom). This perceived direction of rotation switches every few seconds. (C) Turi et al. found that pupil size increased when the black dots appeared to be at the front, and decreased when the white dots appeared to be at the front. The size of this change was correlated with Autism-Spectrum Quotient score.
To track any subjective change in perception, the participants reported how they experienced the rotation of the cylinder, while the researchers measured the pupil size. Although the overall intensity of light (the primary factor influencing pupil size) remained constant, the size of the pupils changed: the pupils became larger when the black dots seemed to be in the front, but became smaller, when the white dots appeared to be in front (Figure 1C).
In some participants, the size of the pupils changed substantially between the black and white phases, while in others the changes were only minimal. Turi et al. revealed that these differences strongly correlated with the Autism-Spectrum Quotient (AQ) scores of the participants (Baron-Cohen et al., 2001). The higher the number of autistic traits reported by an individual in the AQ questionnaire, the stronger the changes in pupil size became. This effect accounted for about half of the variance in AQ scores, which is considerably higher than the sizes of the effects reported for other sensory measures (e.g., Ujiie et al., 2015).
Turi et al. argue that the changes in pupil size reflect how individuals process visual information differently. Some people tend to focus on small, defined areas such as the surface at the front, while others concentrate on the entire cylinder. Consequently, people with a more detailed focus should have more fluctuations in pupil size, because their focus alternates between black and white dots. This observation is tightly linked with the long-standing hypothesis that individuals with autism spectrum disorders tend to focus more on the detail rather than the bigger picture (Happé and Frith, 2006).
The findings of Turi et al. provide compelling evidence that the size of a pupil reflects subjective differences in a way that is highly correlated to the reported autistic traits of the participants. The study provides numerous possibilities to investigate higher-level cognitive processes. This can be particularly valuable when studying individuals who may find it difficult to engage in complex behavioral tasks, such as individuals with minimal language skills or deficits in other cognitive abilities. Pupillometry is clearly a powerful tool for characterizing the individual differences in processing visual information and, potentially, for advancing our understanding of autism spectrum disorders.
References
-
Perception of three-dimensional structure from motionTrends in Cognitive Sciences 2:222–228.https://doi.org/10.1016/S1364-6613(98)01181-4
-
The autism-spectrum quotient (AQ): evidence from Asperger syndrome/high-functioning autism, males and females, scientists and mathematiciansJournal of Autism and Developmental Disorders 31:5–17.https://doi.org/10.1023/A:1005653411471
-
Keeping a large-pupilled eye on high-level visual processingTrends in Cognitive Sciences 19:1–3.https://doi.org/10.1016/j.tics.2014.11.002
-
The weak coherence account: detail-focused cognitive style in autism spectrum disordersJournal of Autism and Developmental Disorders 36:5–25.https://doi.org/10.1007/s10803-005-0039-0
-
Pupillometry: the eyes shed fresh light on the mindCurrent Biology 24:R281–R282.https://doi.org/10.1016/j.cub.2014.02.028
-
Psychophysical magic: rendering the visible 'invisible'Trends in Cognitive Sciences 9:381–388.https://doi.org/10.1016/j.tics.2005.06.012
-
Pupil responses allow communication in locked-in syndrome patientsCurrent Biology 23:R647–R648.https://doi.org/10.1016/j.cub.2013.06.011
-
Factors affecting light-adapted pupil size in normal human subjectsInvestigative Ophthalmology & Visual Science 35:1132–1137.
Article and author information
Author details
Publication history
Copyright
© 2018, Park et al.
This article is distributed under the terms of the Creative Commons Attribution License, which permits unrestricted use and redistribution provided that the original author and source are credited.
Metrics
-
- 1,811
- views
-
- 167
- downloads
-
- 0
- citations
Views, downloads and citations are aggregated across all versions of this paper published by eLife.
Download links
Downloads (link to download the article as PDF)
Open citations (links to open the citations from this article in various online reference manager services)
Cite this article (links to download the citations from this article in formats compatible with various reference manager tools)
Further reading
-
- Neuroscience
As the global population ages, the prevalence of neurodegenerative disorders is fast increasing. This neurodegeneration as well as other central nervous system (CNS) injuries cause permanent disabilities. Thus, generation of new neurons is the rosetta stone in contemporary neuroscience. Glial cells support CNS homeostasis through evolutionary conserved mechanisms. Upon damage, glial cells activate an immune and inflammatory response to clear the injury site from debris and proliferate to restore cell number. This glial regenerative response (GRR) is mediated by the neuropil-associated glia (NG) in Drosophila, equivalent to vertebrate astrocytes, oligodendrocytes (OL), and oligodendrocyte progenitor cells (OPCs). Here, we examine the contribution of NG lineages and the GRR in response to injury. The results indicate that NG exchanges identities between ensheathing glia (EG) and astrocyte-like glia (ALG). Additionally, we found that NG cells undergo transdifferentiation to yield neurons. Moreover, this transdifferentiation increases in injury conditions. Thus, these data demonstrate that glial cells are able to generate new neurons through direct transdifferentiation. The present work makes a fundamental contribution to the CNS regeneration field and describes a new physiological mechanism to generate new neurons.
-
- Developmental Biology
- Neuroscience
During development axons undergo long-distance migrations as instructed by guidance molecules and their receptors, such as UNC-6/Netrin and UNC-40/DCC. Guidance cues act through long-range diffusive gradients (chemotaxis) or local adhesion (haptotaxis). However, how these discrete modes of action guide axons in vivo is poorly understood. Using time-lapse imaging of axon guidance in C. elegans, we demonstrate that UNC-6 and UNC-40 are required for local adhesion to an intermediate target and subsequent directional growth. Exogenous membrane-tethered UNC-6 is sufficient to mediate adhesion but not directional growth, demonstrating the separability of haptotaxis and chemotaxis. This conclusion is further supported by the endogenous UNC-6 distribution along the axon’s route. The intermediate and final targets are enriched in UNC-6 and separated by a ventrodorsal UNC-6 gradient. Continuous growth through the gradient requires UNC-40, which recruits UNC-6 to the growth cone tip. Overall, these data suggest that UNC-6 stimulates stepwise haptotaxis and chemotaxis in vivo.