LEAFY maintains apical stem cell activity during shoot development in the fern Ceratopteris richardii
Figures
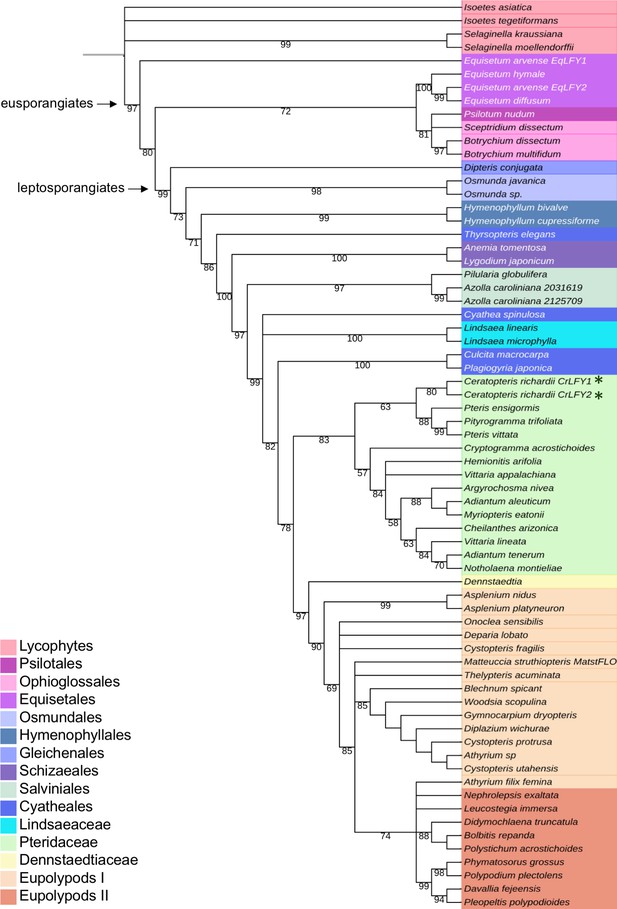
CrLFY1 and CrLFY2 arose from a recent gene duplication event.
Inferred phylogenetic tree from maximum likelihood analysis of 64 LFY amino acid sequences (see Supplementary file 1 for accession numbers) sampled from within the fern lineage plus lycophyte sequences as an outgroup. Bootstrap values are given for each node. The tree shown is extracted from a phylogeny with representative sequences from all land plant lineages (Figure 1—figure supplement 1). The Ceratopteris richardii genome contains no more than two copies of LFY (Figure 1—figure supplement 2; indicated by *). Different taxonomic clades within the fern lineage are denoted by different colours, as shown. The divergence between eusporangiate and leptosporangiate ferns is indicated by arrows.
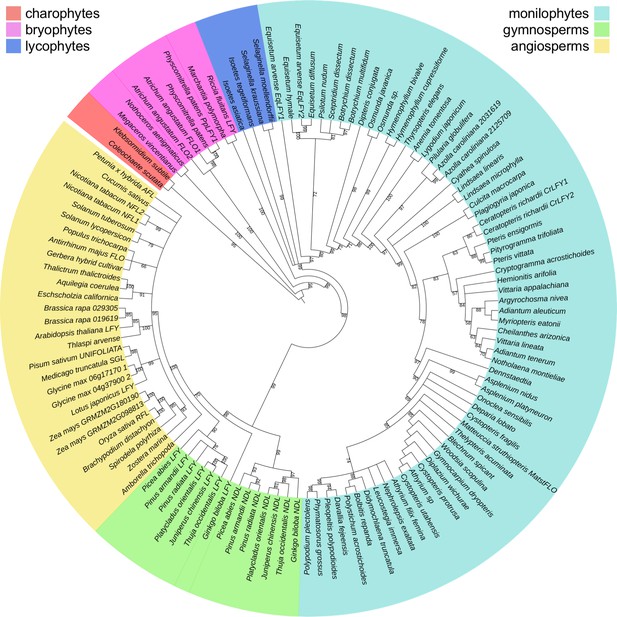
Phylogenetic relationships between LEAFY sequences reflect established relationships within vascular plant lineages.
Inferred phylogenetic tree from maximum likelihood analysis of 120 LFY sequences sampled from across extant land plant lineages (liverworts, mosses, hornworts, lycophytes, monilophytes i.e. ferns and allies, gymnosperms, angiosperms) including algal (charophyte) sequences as an outgroup. Bootstrap values are given for each node. Sequences belonging to each lineage are denoted by different colours, as shown. The higher-order topology between vascular plant lineages (lycophytes, monilophytes, gymnosperms and angiosperms) is consistent with expected relationships; a gene duplication event resulting in LFY and NEEDLY clades in gymnosperms has been identified previously (Sayou et al., 2014); and relationships between bryophyte lineages are consistent with differences in the LFY DNA binding site preference, where hornworts and mosses each differ from the preferred site shared by liverworts and vascular plants (Sayou et al., 2014).
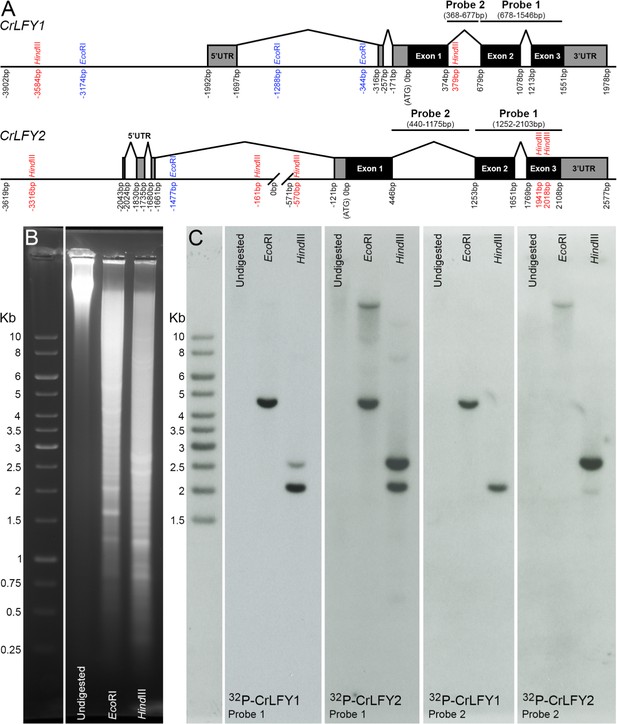
The Ceratopteris genome contains only two copies of LFY.
(A) Deduced gene structure of CrLFY1 and CrLFY2 loci. All positions marked are given relative to the ATG start codon. Hybridization probes used in DNA gel blot analysis and relevant restriction sites (EcoRI, HindIII) are marked. CrLFY1 probe 1 (868bp) and CrLFY2 probe 1 (851bp) share 79% sequence similarity and hybridize to exons 2 + 3 of each gene (comprising the conserved LFY DNA binding domain). As such, both probes should hybridize to all members of the LFY gene family. CrLFY1 probe 2 (309bp) and CrLFY2 probe 2 (735bp) hybridize to intron 1 of each gene copy and share no significant sequence similarity. As such, each probe is expected to hybridize to the specific gene copy. (B, C) Gel blot analysis of wild-type genomic DNA, digested with EcoRI or HindIII, electrophoresed on an ethidium bromide stained gel (B), blotted to nylon membrane and hybridized against different probes (C) as described in (A). EcoRI digestion was predicted to generate single hybridizing fragments for both CrLFY1 and CrLFY2, each spanning both probes with minimum expected fragment sizes of ~2.0 kb and ~3.1 kb, respectively. HindIII digestion was predicted to generate a single CrLFY1 hybridizing fragment recognized by both probes with a minimum size of ~1.6 kb. HindIII digestion was predicted to generate a CrLFY2 fragment of ~2.5 kb hybridizing to probes 1 and 2, a separate fragment with a minimum size of 559 bp overlapped by 85 bp of probe 1 (and so potentially undetectable) plus an undetectable fragment of 11 bp. The hybridization patterns observed (C) are consistent with these predictions, with the exon probes cross-hybridizing to predicted fragments of both gene copies (but not to any additional gene fragments) and the intron probes primarily hybridizing to the respective specific gene copy.
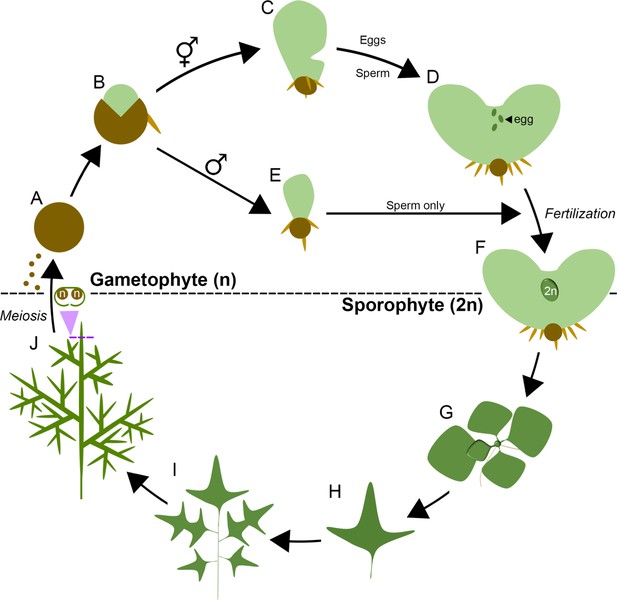
The lifecycle of Ceratopteris richardii.
Ceratopteris propagates in the haploid gametophyte phase of its lifecycle (n) through single-celled spores (A) On spore germination (B) a two-dimensional photosynthetic thallus develops into one of two sexes, a default hermaphrodite (C) which produces eggs and sperm (D) or a hormone-induced male that produces sperm only (E). Eggs are retained on the hermaphrodite thallus, and fertilization results in the development of a diploid (2n) embryo on the gametophyte (F), initiating the sporophyte phase of the lifecycle. The sporophyte establishes a vegetative shoot that initiates leaflike lateral organs (fronds) and roots from its apex (G). The first fronds produced are simple but later fronds become increasingly lobed and dissected (H, I). The sporophyte undergoes a reproductive phase-change and subsequent fronds generate haploid spores by meiosis on their undersides (J), enclosed in a morphologically-distinct curled lamina. Mature spores are dispersed to restart the lifecycle.
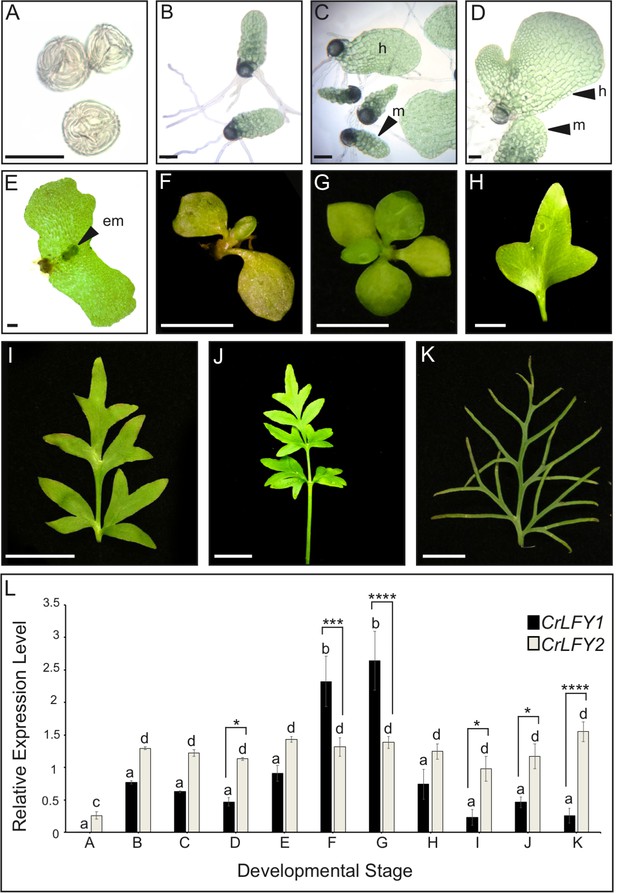
CrLFY1 and CrLFY2 are differentially expressed during the Ceratopteris lifecycle.
(A-K) Representative images of the developmental stages sampled for expression analysis in (L). Imbibed spores (A); populations of developing gametophytes harvested at 5 (B, C) and 8 (D) days after spore-sowing (DPS), comprising only males (B) or a mixture of hermaphrodites (h) and males (m) (C, D); fertilized gametophyte subtending a developing sporophyte embryo (em) (E); whole sporophyte shoots comprising the shoot apex with 3 (F) or five expanded entire fronds attached (G); individual vegetative fronds demonstrating a heteroblastic progression in which frond complexity increases through successive iterations of lateral outgrowths (pinnae) (H–J); complex fertile frond with sporangia on the underside of individual pinnae (K). Scale bars = 100 um (A–E), 5 mm (F–H), 20 mm (I–K). (L) Relative expression levels of CrLFY1 and CrLFY2 (normalized against the housekeeping genes CrACTIN1 and CrTBP) at different stages of development. n = 3; Error bars = standard error of the mean (SEM). Pairwise statistical comparisons (ANOVA followed by Tukey’s multiple comparisons test– Supplementary file 4) found no significant difference in CrLFY2 transcript levels between any gametophyte or sporophyte tissues sampled after spore germination (p>0.05) and no significant difference between CrLFY1 and CrLFY2 transcript levels during early gametophyte development (p>0.05) (B, C). Differences between CrLFY1 and CrLFY2 transcript levels were significant in gametophytes at 8 DPS (p<0.05) (D). CrLFY1 transcript levels were significantly higher in whole young sporophytes (F) and vegetative shoots (G) compared to isolated fronds (H–K) (p<0.05). CrLFY1 transcript levels in whole sporophytes and shoots were greater than CrLFY2, whereas in isolated fronds CrLFY1 transcript levels were consistently lower than CrLFY2 (p<0.05). Asterisks denote significant difference (*, p<0.05; **, p<0.01, ***, p<0.001; ****, p<0.0001) between CrLFY1 and CrLFY2 transcript levels (Sidak’s multiple comparisons test) within a developmental stage. Letters denote significant difference (p<0.05) between developmental stages for CrLFY1 or CrLFY2 (Tukey’s test). Groups marked with the same letter are not significantly different from each other (p>0.05). Statistical comparisons between developmental stages were considered separately for CrLFY1 and CrLFY2. The use of different letters between CrLFY1 and CrLFY2 does not indicate a significant difference.
-
Figure 3—source data 1
CrLFY qRT-PCR ontogenic expression data
- https://doi.org/10.7554/eLife.39625.008
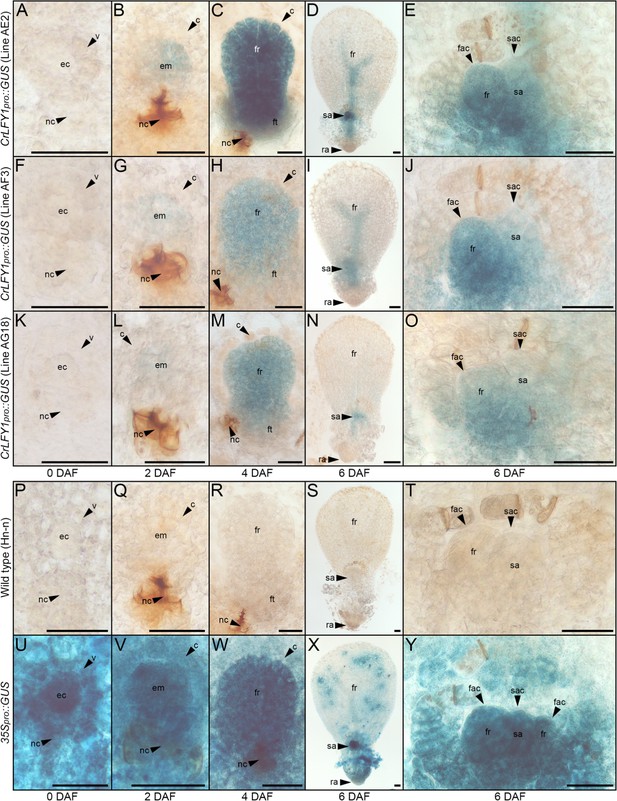
The CrLFY1 promoter drives reporter gene expression in proliferating tissues of the developing Ceratopteris embryo.
(A–Y) GUS activity detected as blue staining in developing embryos of three independent CrLFY1pro::GUS transgenic reporter lines (A–O), a representative negative wild-type control line (P–T) and a representative positive 35Spro::GUS control line (U–Y). Tissues are shown prior to fertilization (A, F, K, P, U), or 2 (B, G, L, Q, V), 4 (C, H, M, R, W), and 6 (D, I, N, S, X) days after fertilization (DAF). In CrLFY1pro::GUS lines, GUS activity first became visible within the first few divisions of embryo development (but not in surrounding gametophyte tissues) at 2 DAF (B, G, L) and was expressed in cells of the embryo frond as it proliferated (C, H, M). GUS activity was visible in the shoot apex and in frond vascular tissue at 6 DAF (D, I, N), with staining in the shoot apical cell (sac), subtending shoot apex tissues and newly-initiated fronds, including the frond apical cell (fac) (E, J, O). No GUS activity was detected in wild-type samples (P–T), whereas the majority of cells in the constitutively expressing 35Spro::GUS samples stained blue (U–Y). Embryos develop on the surface of the gametophyte thallus when an egg cell (ec) within the archegonium (which comprises a venter (v) and neck cells (nc) to allow sperm entry) are fertilized. After fertilization, the venter forms a jacket of haploid cells known as the calyptra (c) that surrounds the diploid embryo (em). Cell fates in the embryo (embryo frond (fr), embryo foot (ft), root apex (ra) and shoot apex (sa)) are established at the eight-celled stage (Johnson and Renzaglia, 2008), which is around 2 DAF under our growth conditions. Embryogenesis is complete at 6 DAF, after which fronds arise from the shoot apex. Scale bars = 50 μm.
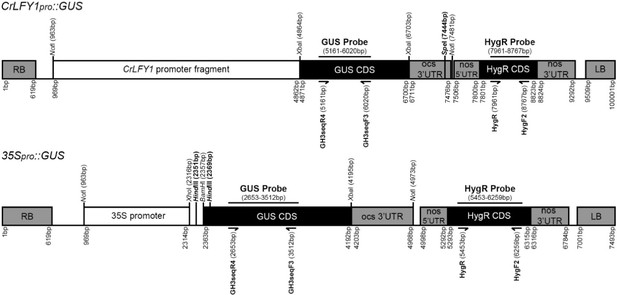
Schematic of CrLFY1pro::GUS and 35Spro::GUS constructs.
Hybridization probes for the GUS and hygromycin resistance (HygR) genes, plus the position of the SpeI and HindIII restriction sites used for DNA gel blot analysis (Figure 4—figure supplement 2) are indicated. Restriction sites used in plasmid construction (see Materials and methods) are also indicated. All nucleotide positions are given relative to the start of the T-DNA right border (RB). Primer sequences are listed in the Key Resources Table.
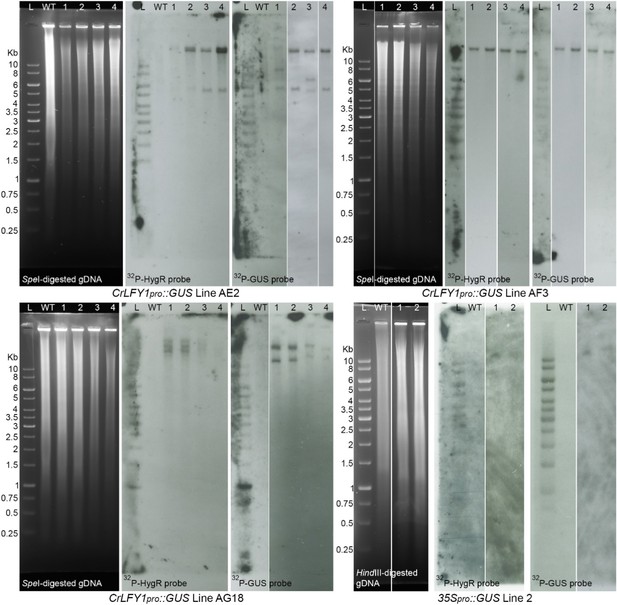
DNA gel blot analysis of CrLFY1pro::GUS and 35Spro::GUS transgenic lines.
CrLFY1pro::GUS lines AE2, AF3, and AG18 were regenerated from three separate bombardments and so are necessarily independent of one another. Genomic DNA was extracted from four T1 sporophytes (arising from the free fertilization of T1 gametophytes) within each line, digested with SpeI and separated on an electrophoresis gel. Genomic DNA from two T1 sporophytes of 35Spro::GUS line two was digested with HindIII. Gel blot analysis of both constructs was performed with the same two probes, hybridizing to the GUS CDS and hygromycin resistance (HygR) CDS, respectively (Figure 4—figure supplement 1). For each full-length CrLFY1pro::GUS T-DNA insertion present in the genome a single insert is predicted to result in unlinked hybridization fragments with minimum sizes of ~2.5 kb (HygR) and ~6 kb (GUS). For 35Spro::GUS, a single fragment with a minimum size of ~5.1 kb is predicted per insertion event for both probes (see Figure 4—figure supplement 1). Gel blot results indicate the presence of one full-length T-DNA insertion in 35Spro::GUS line 2. Based on the hybridization fragments obtained, CrLFY1pro::GUS line AE2 contains two potentially full-length insertions of the CrLFY1pro::GUS cassette and 1–2 insertions of partial fragments, AF3 contains a single potential full length insertion and AG18 contains two potential full length insertions.
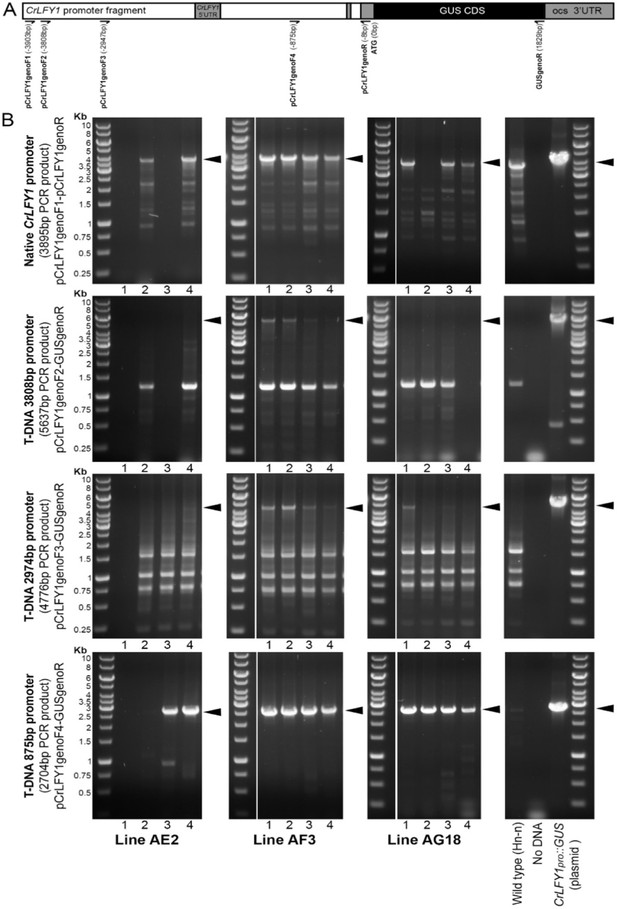
PCR analysis of CrLFY1pro::GUS T1 lines identified full-length or near full-length CrLFY1 promoter sequences in T-DNA insertions.
(A) Schematic of the CrLFY1pro::GUS construct marking binding sites of PCR primers used in (B). All positions are given relative to the GUS ATG. Primer sequences are listed in the Key Resources Table. (B) PCR was performed on genomic DNA from the same four individual T1 sporophytes within each line investigated by gel blot analysis (see Figure 4—figure supplement 2). PCR reactions were performed to amplify the native 3.9 kb CrLFY1 promoter as a positive control (row 1) and to amplify T-DNA specific products (rows 2–4) containing the GUS CDS and different lengths of contiguous CrLFY1 promoter sequence (see A). Black arrowheads mark the expected size of the target PCR product in each reaction. PCR analysis identified at least one full-length GUS CDS with ~3.8 kb of CrLFY1 promoter in line AF3 (row 2). Faint PCR products indicate that line AG18 and AE2 probably contain a full-length GUS CDS plus ~3 kb of CrLFY1 promoter sequence (row 3). All lines carry a GUS CDS plus a minimum CrLFY1 promoter length of 875 bp (row 4).
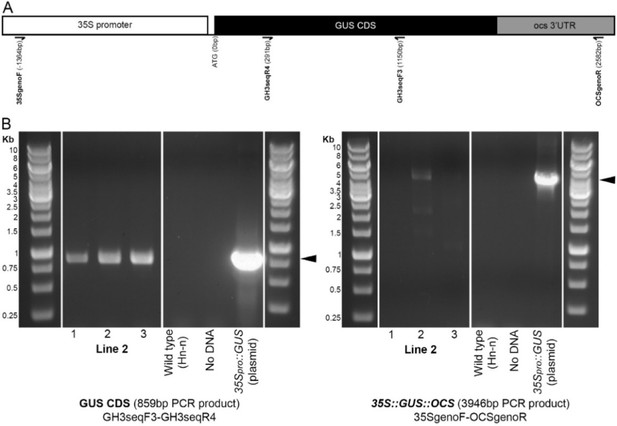
PCR analysis of 35Spro::GUS positive control line identified a full-length 35Spro::GUS insertion.
(A) Schematic of the 35Spro::GUS construct marking binding sites of genotyping primers used in (B). All positions shown are relative to the GUS ATG. Primer sequences are listed in the Key Resoucres Table. (B) PCR was performed on genomic DNA extracted from three T1 individuals, including the two investigated by gel blot analysis (Figure 4—figure supplement 2). T-DNA specific reactions were performed to amplify the GUS CDS (left) previously identified by gel blot analysis (Figure 4—figure supplement 2) using the same primers as the gel-blot probe, and a PCR product spanning almost the full length of the 35S::GUS::OCS construct (right). Black arrowheads mark the expected size of the target PCR product in each reaction. PCR results indicate the presence of a full length 35Spro::GUS T-DNA in this line.
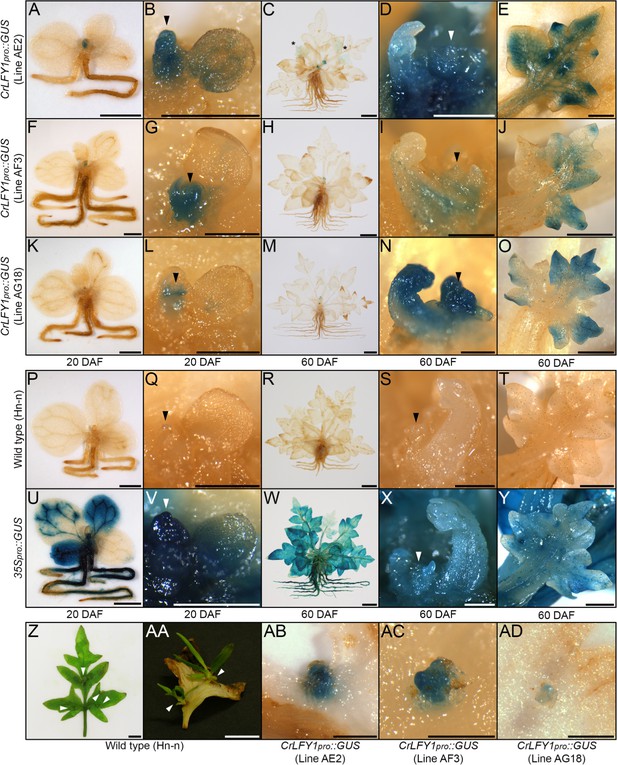
The CrLFY1 promoter drives reporter gene expression in proliferating shoot tissues of the Ceratopteris sporophyte.
(A–Y) GUS activity detected as blue staining in post-embryonic sporophytes from three independent CrLFY1pro::GUS transgenic reporter lines (A–O), negative wild-type controls (P–T) and positive 35Spro::GUS controls (U–Y). Sporophytes were examined at 20 DAF (A, B, F, G, K, L, P, Q, U, V) and 60 DAF (C–E, H–J, M–O, R–T, W–Y). GUS staining patterns are shown for whole sporophytes (A, C, F, H, K, M, P, R, U, W), shoot apices (arrowheads) (B, D, G, I, L, N, Q, S, V, X) and developing fronds (E, J, O, T, Y). In CrLFY1pro::GUS sporophytes at 20 DAF (producing simple, spade-like fronds) GUS activity was restricted to the shoot apex (A, F, K) and newly-initiated frond primordia, with very low activity in expanded fronds (B, G, L). In CrLFY1pro::GUS sporophytes at 60 DAF (producing complex, highly dissected fronds) GUS activity was similarly seen in the apex (C, H, M), but persisted for longer during frond development. Activity was initially detected throughout the frond primordium (D, I, N), before becoming restricted to actively proliferating areas of the lamina (E, J, O). Scale bars = 2 mm (A, F, K, P, U), 500 μm (B, D, G, I, L, N, Q, S, V, X) 10 mm (C, H, M, R, W), 1 mm (E, J, O, T, Y). *=GUS staining in maturing frond. GUS staining patterns were the same in leaves formed after the reproductive transition (Figure 5—figure supplement 1). (Z-AD) Fronds can initiate de novo shoots (white arrowheads) from marginal tissue between existing frond pinnae (Z, AA). GUS activity was detected in emerging de novo shoot apices on CrLFY1pro::GUS fronds (AB–AD). Scale bars = 10 mm (Z, AA), 500 μm (AB–AD).
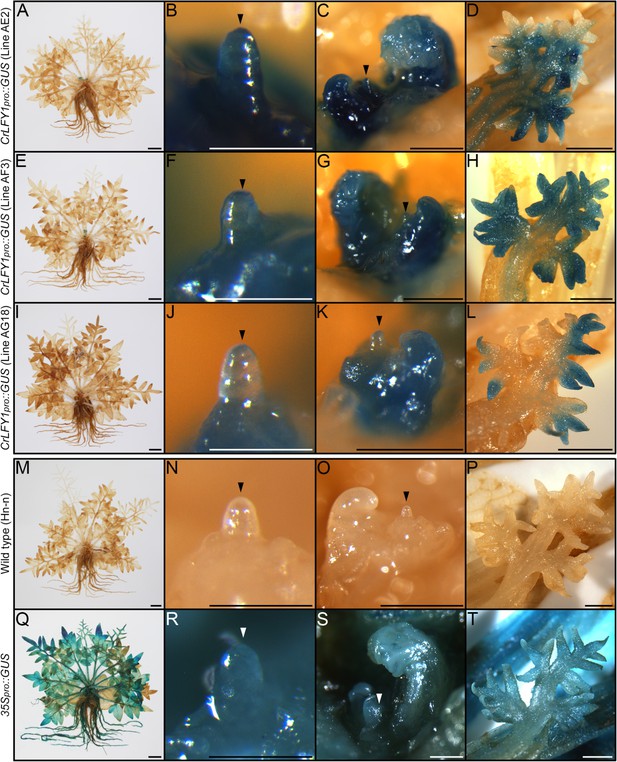
CrLFY1pro::GUS expression patterns are similar in Ceratopteris shoots before and after reproductive phase change.
GUS activity detected as blue staining in sporophytes producing fronds with spore-bearing morphology (narrowing and elongation of pinnae) from three independent CrLFY1pro::GUS transgenic reporter lines (A–L); 110–124 DAF), negative wild-type controls (M–P); 113 DAF) and positive 35Spro::GUS controls (Q–T); 110 DAF). Staining patterns were consistent between the three independent CrLFY1pro::GUS transgenic lines (A, E, I), and were similar to those seen at 60 DAF (Figure 4C, (H,M). GUS activity was observed throughout the shoot apex (B, F, J) and in recently-emerged frond primordia (C, G, K). Activity persisted later in frond development, becoming restricted to developing pinnae (D, H, L). GUS staining was lost from fronds prior to maturity (A, E, I). No endogenous GUS activity was detected in wild-type controls (M–P) whereas activity was detected throughout all non-senescent tissues in the 35Spro::GUS line (Q–T).
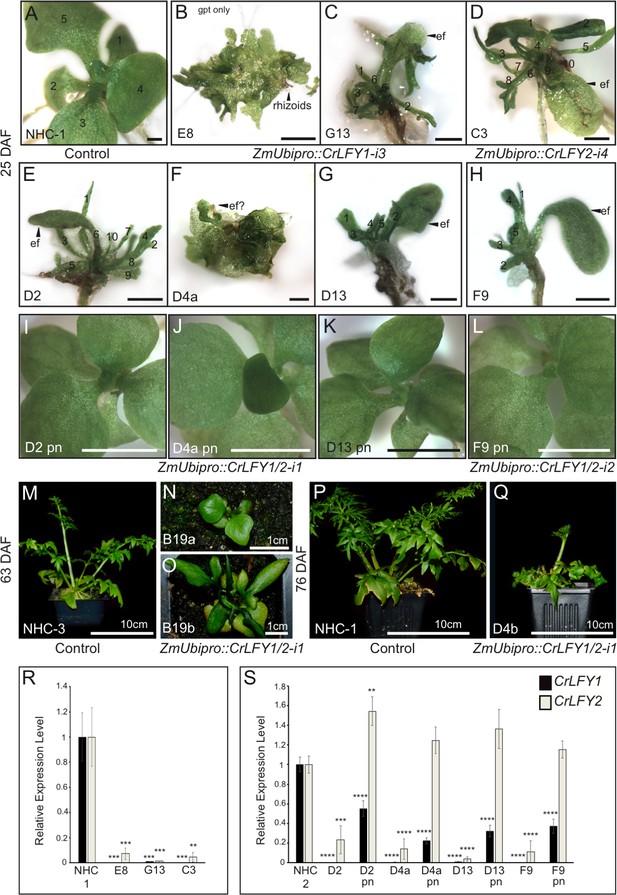
Suppression of CrLFY expression causes early termination of the Ceratopteris sporophyte shoot apex.
(A-L) Sporophyte phenotype 25 days after fertilization (DAF) in no hairpin control, NHC-1 (A) and transgenic lines carrying RNAi constructs against CrLFY1 (ZmUbipro::CrLFY1-i3) (B, C), CrLFY2 (ZmUbipro::CrLFY1-i4) (D) and both CrLFY1 and CrLFY2 (ZmUbipro::CrLFY1/2-i1 and ZmUbipro::CrLFY1/2-i2) (E–L). In some lines, both aborted and phenotypically normal sporophytes were identified (compare E and I; F and J; G and K; H and L). The presence of the RNAi transgene in phenotypically normal sporophytes was validated by genotyping (Figure 6—figure supplement 5). Scale bars = 1 mm (A–H), 5 mm (I–L). (M–Q) Sporophyte phenotype of two no hairpin control (NHC-3 and NHC-1) (M, P) and two ZmUbipro::CrLFY1/2-i1 (N, O) lines at 63 (M–O) and 76 (P,Q) DAF. (R, S) qRT-PCR analysis of CrLFY1 and CrLFY2 transcript levels (normalized against the averaged expression of reference genes CrACTIN1 and CrTBP) in the sporophytes of the RNAi lines shown in (A–L). Transcript levels are depicted relative to no hairpin controls (NHC-1or −3), n = 3, error bars = standard error of the mean (SEM). CrLFY1 and CrLFY2 expression levels were significantly reduced compared to controls (p<0.01 or less) in all transgenic lines where sporophyte shoots undergo early termination (A–H), but in phenotypically normal (pn) sporophytes segregating in the same lines (I–L), only CrLFY1 transcript levels were reduced (p<0.0001). CrLFY2 transcript levels in pn sporophytes were not significantly lower than in controls. Asterisks denote level of significant difference from controls (**p<0.01, ***p<0.001; ****p<0.0001).
-
Figure 6—source data 1
CrLFY RNAi lines qRT-PCR expression data
- https://doi.org/10.7554/eLife.39625.023
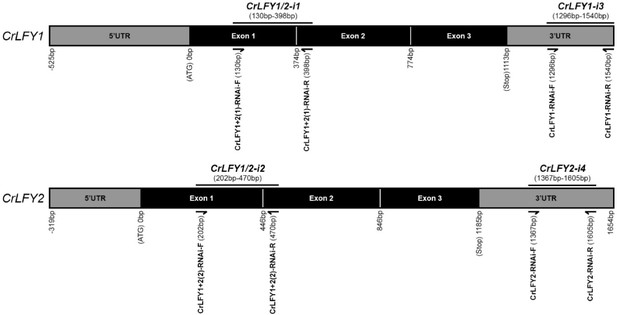
Positions of CrLFY RNAi target sequences.
Schematic of CrLFY1 and CrLFY2 transcripts, showing recognition sequences used in RNAi constructs (black bars). 5’ and 3’ untranslated regions (UTRs) are marked by grey boxes, coding sequence (CDS) by black boxes, with exons as indicated. Positions are given relative to the translational start codon of each transcript. Four RNAi constructs were generated Two of these (ZmUbipro::CrLFY1/2-i1 and ZmUbipro::CrLFY1/2-i2) targeted both CrLFY1 and CrLFY2 using conserved coding sequence amplified from CrLFY1 (ZmUbipro::CrLFY1/2-i1) or CrLFY2 (ZmUbipro::CrLFY1/2-i2). The two remaining constructs (ZmUbipro::CrLFY1-i3 and ZmUbipro::CrLFY2-i4) incorporate target sequence amplified from the 3’UTR region of CrLFY1 and CrLFY2, respectively. The position of primers used in target sequence amplification are shown. Primer sequences are supplied in the Key Resources table.
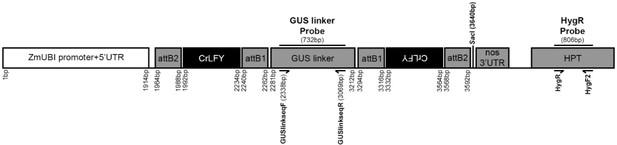
Generalized schematic of CrLFY RNAi constructs.
Each RNAi construct carries inverted repeats of CrLFY-derived sequence (see Figure 6—figure supplement 1 and Supplementary file 5) to generate a hairpin bridged by a linker sequence derived from the GUS CDS (Miki and Shimamoto, 2004). The positions given are relative to the start of the maize ubiquitin promoter (ZmUbipro) that is driving RNAi expression, and are shown for ZmUbipro::CrLFY1-i3, with the length of the RNAi target sequence varying between the four different constructs (see Figure 6—figure supplement 1). The sites of hybridization against probes for the GUS linker and hygromycin resistance marker (HygR) are shown. The position of a SacI restriction site (used in gel blot analysis, see Figure 6—figure supplement 3) is indicated. No SacI sites are present in any CrLFY target sequence used. The primers used in probe amplification are given in the Key Resources Table.
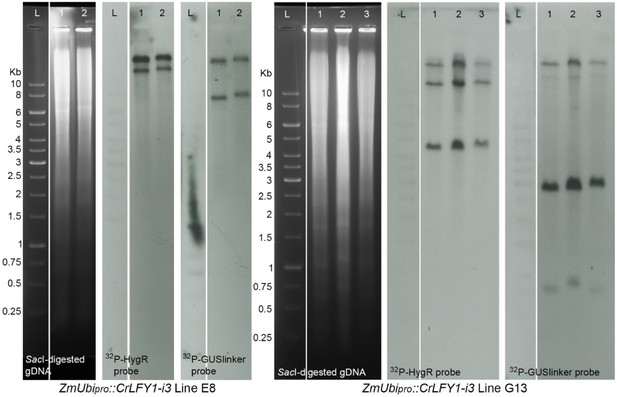
Gel blot analysis of ZmUbipro::CrLFY1-i3 T1 transgenic lines.
ZmUbipro::CrLFY1-i3 lines E8 and G13 were regenerated from two separate bombardments and so are necessarily independent of one another. Genomic DNA was extracted from four T1 sporophytes (arising from the free fertilization of T1 gametophytes) within each line, digested with SpeI and separated on an electrophoresis gel. Gel blot analysis of both constructs was performed with probes hybridizing either to the GUS linker of the RNAi hairpin or to the hygromycin resistance (HygR) CDS (Figure 6—figure supplement 2). For each full-length ZmUbipro::CrLFY1-i3 T-DNA insertion present in the genome a single insert is predicted to result in unlinked hybridization fragments with minimum sizes of ~1 kb (HygR) or ~3.6 kb (GUS linker). Based on the hybridization fragments obtained, line E8 carries two full-length insertions of the CrLFY1 RNAi cassette and line G13 carries two full-length insertions plus two additional partial insertions.
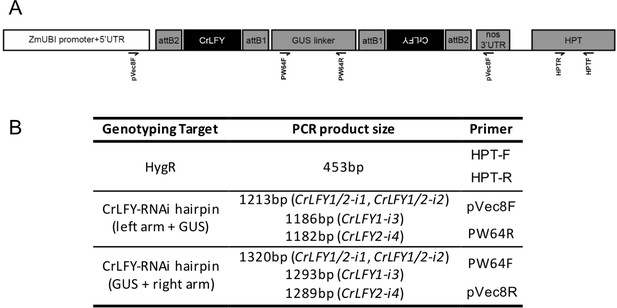
Binding site of CrLFY RNAi genotyping PCR primers.
(A) Generalized schematic of CrLFY RNAi T-DNA with the relative position of primers used in genotyping PCR (see Figure 6—figure supplement 5, Figure 7—figure supplement 1) marked. The primer binding sites are common to all four RNAi constructs, with the size of the hairpin-containing PCR products varying due to the insertion of different CrLFY target sequences. (B) Primer combinations for each genotyping reaction and expected PCR product sizes for each CrLFY RNAi construct. Primer sequences are listed in the Key Resources Table.
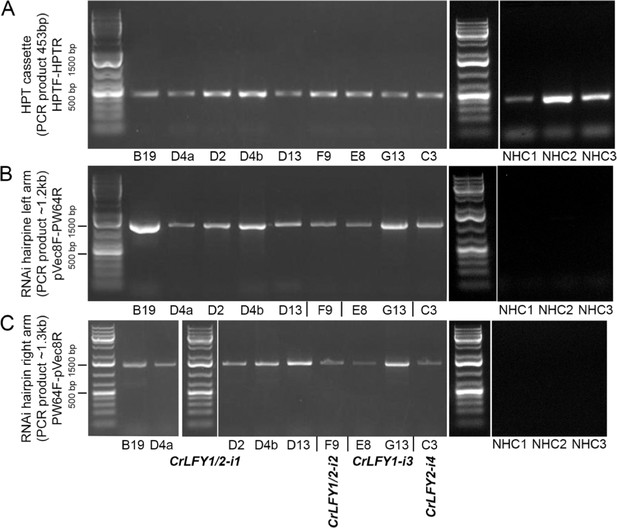
Genotyping PCR confirms the presence of CrLFY RNAi T-DNA in transgenic lines and the absence of the RNAi hairpin in no hairpin control lines.
PCR was performed on genomic DNA extracted from T1 sporophytes pre-selected for antibiotic resistance. The presence of the HPT cassette (A) was confirmed in all lines by PCR. The presence or absence of both arms of the RNAi hairpin (B, C) were confirmed in each line by PCR, and where sequenced the amplified products were as expected.
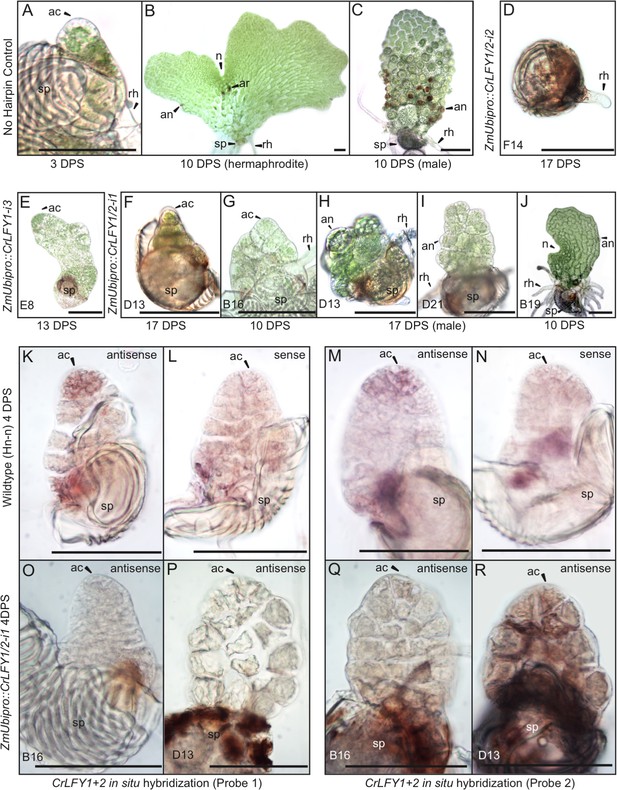
Suppression of CrLFY expression causes early termination of the Ceratopteris gametophyte apical cell.
(A–C) In no hairpin control lines, the gametophyte established a triangular apical cell (ac) shortly after spore (sp) germination (A). Divisions of the apical cell established a photosynthetic thallus in both hermaphrodite and male gametophytes. At 10 days post spore sowing (DPS) both gametophyte sexes were approaching maturity, with the hermaphrodite (B) having formed a chordate shape from divisions at a lateral notch meristem (n) and having produced egg-containing archegonia (ar), sperm-containing antheridia (an), and rhizoids (rh). The male (C) had a more uniform shape with antheridia across the surface. These phenotypes were identical to wild-type. (D–J) When screened at 10–17 DPS, gametophytes from multiple RNAi lines (as indicated) exhibited developmental arrest, mostly associated with a failure of apical cell activity. Arrest occurred at various stages of development from failure to specify an apical cell, resulting in only a rhizoid being produced and no thallus (D) through subsequent thallus proliferation (E–I). Gametophyte development in one line progressed to initiation of the notch meristem but overall thallus size was severely reduced compared to wild-type (J). (K–R) In situ hybridization with antisense probes detected CrLFY transcripts in the apical cell and immediate daughter cells of wild-type gametophytes at 4 DPS (K, M). No corresponding signal was detected in controls hybridized with sense probes (L, N). In the arrested gametophytes of two ZmUbipro::CrLFY1/2-i1 lines CrLFY transcripts could not be detected (O–R), and transgene presence was confirmed (Figure 7—figure supplement 1). Scale bars = 100 μm.
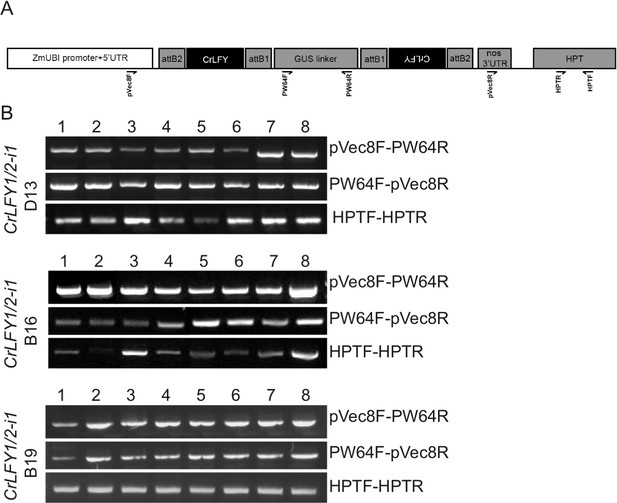
Gametophytes exhibiting developmental arrest were transgenic.
A. Generalized schematic of CrLFY RNAi T-DNA with the relative position of primers used in genotyping PCR marked. Primer sequences and expected PCR product sizes for each CrLFY RNAi construct are given in Figure 6—figure supplement 4. Genotyping PCR was conducted on DNA extracted from single gametophytes exhibiting developmental arrest at 10 DPS in three ZmUbipro::CrLFY1/2-i1 lines. DNA from all arrested individuals amplified positive bands for the two hairpin arms (pVec8F-PW64R and PW64F-pVec8R) and for the hygromycin resistance marker (HPTF-HPTR).
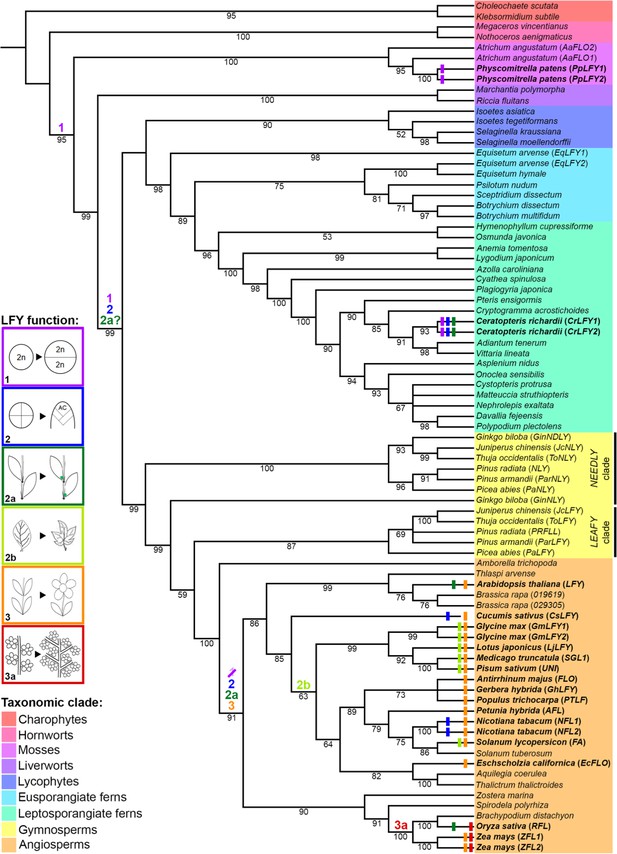
Evolutionary trajectory of LFY function.
The phylogeny was reconstructed from selected LFY protein sequences representing all extant embryophyte lineages (as highlighted) and the algal sister-group. Coloured bars at the terminal branches represent different developmental functions of LFY determined from functional analysis in those species (see Supplementary file 8 for references). Coloured numbers indicate the putative points of origin of different functions inferred from available data points across the tree. 1, cell division within the sporophyte zygote; 2, maintenance of indeterminate cell fate in vegetative shoots through proliferation of one or more apical cells (AC); 2a, maintenance of indeterminate cell fate in vegetative lateral/axillary apices; 2b, maintenance of indeterminate cell fate in the margins of developing lateral organs (compound leaves); 3, specification of floral meristem identity (determinate shoot development producing modified lateral organs) and shoot transition to the reproductive phase; 3a, maintenance of indeterminate cell fate in inflorescence lateral/branch meristems (in place of floral meristem fate).
Tables
Summary of CrLFY RNAi transgenic lines and their phenotypic characterization.
Transgenic lines exhibited gametophytic developmental arrest and/or sporophyte shoot termination at varying stages of development. ‘+’ indicates that a particular line was phenotypically normal at the developmental stage indicated, ‘−’ indicates that development had arrested at or prior to this stage. In lines marked ‘+/-’ the stage at which developmental defects occurred varied between individuals within the line, and at least some arrested individuals were identified at the stage indicated. The five ZmUbipro::CrLFY1/2-i1 lines shown were generated from three rounds of transformation, the pairs of lines B16 and B19 and D2 and D4 potentially arising from the same transformation event. The no hairpin control lines NHC-2 (F3) and NHC-3 (F4) may similarly have arisen from a single transformation event. In all other cases, each transgenic line arose from a separate round of transformation and so must represent independent T-DNA insertions.
RNAi transgene | Line | Transfor-mation replicate | Gametophyte phase | Sporophyte phase | |||||||
---|---|---|---|---|---|---|---|---|---|---|---|
Spore germin-ation | AC-based growth | Notch meristem-based growth | % arrested | Embryo | Shoot apex initiated | Simple frond | Complex frond | % arrested | |||
ZmUBIpro::CrLFY1/2-i1 | B16 | 1 | + | - | - | 99.86 | + | + | - | - | <5% |
ZmUBIpro::CrLFY1/2-i1 | B19 | 1 | + | - | - | 50.00 | + | + | + | - | <5% |
ZmUBIpro::CrLFY1/2-i1 | D13 | 2 | + | - | - | 99.80 | + | + | - | - | <5% |
ZmUBIpro::CrLFY1/2-i1 | D2 | 3 | + | + | + | 0.00 | + | + | - | - | <5% |
ZmUBIpro::CrLFY1/2-i1 | D4 | 3 | + | + | + | 0.00 | + | + | + | + | <5% |
ZmUBIpro::CrLFY1/2-i2 | F9 | 4 | + | - | - | 0.00 | + | + | - | - | <5% |
ZmUBIpro::CrLFY1/2-i2 | F14 | 5 | - | - | - | 100.00 | - | - | - | - | 0 |
ZmUBIpro::CrLFY1-i3 | E8 | 6 | + | +/- | +/- | 100.00 | - | - | - | - | 0 |
ZmUBIpro::CrLFY1-i3 | G13 | 7 | + | + | + | 0.00 | + | + | - | - | <5% |
ZmUBIpro::CrLFY2-i4 | C3 | 8 | + | + | + | 0.00 | + | + | - | - | <5% |
NHC-1 (control) | D20 | 9 | + | + | + | 0.00 | + | + | + | + | 0 |
NHC-2 (control) | F3 | 10 | + | + | + | 0.00 | + | + | + | + | 0 |
NHC-3 (control) | F4 | 10 | + | + | + | 0.00 | + | + | + | + | 0 |
Reagent type (species) or resource | Designation | Source or reference | Identifiers | Additional information |
---|---|---|---|---|
Gene (Ceratopteris richardii) | CrLEAFY1 (CrLFY1) | Himi et al. (2001), PMID:11675598; This paper | NCBI:AB049974.2; NCBI:MH841970 | cDNA only; ORF plus contiguous promoter |
Gene (C. richardii) | CrLEAFY2 (CrLFY2) | Himi et al. (2001), PMID:11675598; This paper | NCBI:AB049975.2; NCBI:MH841971 | cDNA only; ORF plus contiguous promoter |
Strain, strain background (C. richardii) | Wild type (Hn-n) | Warne and Hickok, 1987, PMID:16665325 | ||
Genetic reagent (C. richardii) | CrLFY1/2-i1 | This paper | C. richardii transgenic line; RNAi knockdown of CrLFY1 and CrLFY2 expression. | |
Genetic reagent (C. richardii) | CrLFY1/2-i2 | This paper | C. richardii transgenic line; RNAi knockdown of CrLFY1 and CrLFY2 expression. | |
Genetic reagent (C. richardii) | CrLFY1-i3 | This paper | C. richardii transgenic line; RNAi knockdown of CrLFY1 expression. | |
Genetic reagent (C. richardii) | CrLFY2-i4 | This paper | C. richardii transgenic line; RNAi knockdown of CrLFY2 expression. | |
Genetic reagent (C. richardii) | CrLFY1pro::GUS | This paper | C. richardii transgenic line; CrLFY1pro::GUS reporter. | |
Genetic reagent (C. richardii) | 35Spro::GUS | This paper | C. richardii transgenic line; 35Spro::GUS reporter. | |
Recombinant DNA reagent | CrLFY1pro | This paper | NCBI:MH841970 | CrLFY1 5' genomic fragment; Figure 1—figure supplement 2 |
Recombinant DNA reagent | CrLFY2pro fragment 1 | This paper | NCBI:MH841971 | CrLFY2 5' genomic fragment; Figure 1—figure supplement 2 |
Recombinant DNA reagent | CrLFY2pro fragment 2 | This paper | CrLFY2 5' genomic fragment; Figure 1—figure supplement 2; Supplementary file 7 | |
Recombinant DNA reagent | GUS | Ulmasov, 1997, PMID:9401121 | Β-Glucuronidase (GUS) coding sequence | |
Recombinant DNA reagent | pANDA (RNAi vector) | Miki and Shimamoto (2004), PMID:15111724 | ||
Recombinant DNA reagent | pCR4-TOPO (Cloning vector) | Invitrogen | Thermo Scientific: K457502 | |
Recombinant DNA reagent | pDONR207 (Gateway vector) | Invitrogen | ||
Recombinant DNA reagent | pBOMBER (Binary vector) | Plackett et al. (2015), PMID:26146510 | NCBI:MH841969 | Modified pART27 (PMID:1463857); Hygromycin resistance antibiotic selection marker |
Recombinant DNA reagent | pART7 (Cloning vector) | Gleave 1992, PMID:1463857 | ||
Recombinant DNA reagent | ZmUbipro::CrLFY1/2-i1-pANDA | This paper | RNAi construct targeting CrLFY1 and CrLFY2; Figure 6—figure supplement 1; Figure 6—figure supplement 2 | |
Recombinant DNA reagent | ZmUbipro::CrLFY1/2-i2-pANDA | This paper | RNAi construct targeting CrLFY1 and CrLFY2; Figure 6—figure supplement 1; Figure 6—figure supplement 2 | |
Recombinant DNA reagent | ZmUbipro::CrLFY1-i3-pANDA | This paper | RNAi construct targeting CrLFY1; Figure 6—figure supplement 1; Figure 6—figure supplement 2 | |
Recombinant DNA reagent | ZmUbipro::CrLFY2-i4-pANDA | This paper | RNAi construct targeting CrLFY2; Figure 6—figure supplement 1; Figure 6—figure supplement 2 | |
Recombinant DNA reagent | CrLFY1pro::GUS-pBOMBER | This paper | GUS reporter construct, CrLFY1; Figure 4—figure supplement 1 | |
Recombinant DNA reagent | 35Spro::GUS-pBOMBER | This paper | GUS reporter construct, 35S control; Figure 4—figure supplement 1 | |
Recombinant DNA reagent | CrLFY1 in situ probe (antisense) | This paper | In situ hybridisation probe; Supplementary file 6 | |
Recombinant DNA reagent | CrLFY1 in situ probe (sense) | This paper | In situ hybridisation probe; Supplementary file 6 | |
Recombinant DNA reagent | CrLFY2 in situ probe (antisense) | This paper | In situ hybridisation probe; Supplementary file 6 | |
Recombinant DNA reagent | CrLFY2 in situ probe (sense) | This paper | In situ hybridisation probe; Supplementary file 6 | |
Recombinant DNA reagent | 32P-CrLFY1 probe 1 | This paper | DNA gel blot probe for CrLFY1; Figure 1—figure supplement 2 | |
Recombinant DNA reagent | 32P-CrLFY1 probe 2 | This paper | DNA gel blot probe for CrLFY1; Figure 1—figure supplement 2 | |
Recombinant DNA reagent | 32P-CrLFY2 probe 1 | This paper | DNA gel blot probe for CrLFY2; Figure 1—figure supplement 2 | |
Recombinant DNA reagent | 32P-CrLFY2 probe 1 | This paper | DNA gel blot probe for CrLFY2; Figure 1—figure supplement 2 | |
Recombinant DNA reagent | 32P-HygR probe | Plackett et al. (2014), PMID:24623851 | DNA gel blot probe, T-DNA specific; Figure 4—figure supplement 1 | |
Recombinant DNA reagent | 32P-GUS probe | Plackett et al. (2014), PMID:24623851 | DNA gel blot probe, T-DNA specific; Figure 4—figure supplement 1 | |
Recombinant DNA reagent | 32P-GUSlinker probe | This paper | DNA gel blot probe, T-DNA specific; Figure 6—figure supplement 2 | |
Sequence-based reagent | CrLFY1ampF | This paper | ORF amplification, CrLFY1: 5'-ATGGATGTCTCT TTATTGCCAC-3' | |
Sequence-based reagent | CrLFY1ampR | This paper | ORF amplification, CrLFY1: 5'-TCAATCATAGATGC AGCTATCACTG-3' | |
Sequence-based reagent | CrLFY1ampF | This paper | ORF amplification, CrLFY2: 5'-ATGTTCCGATGG GAACAAAG-3' | |
Sequence-based reagent | CrLFY1ampR | This paper | ORF amplification, CrLFY2: 5'-TTATTCATAGCT GCAGCTGTC-3' | |
Sequence-based reagent | CrLFY1invF | This paper | Inverse PCR, CrLFY1: 5'-CTATGGAGTAC GAAGCACCAC-3' | |
Sequence-based reagent | CrLFY1invF2 | This paper | Inverse PCR, CrLFY1: 5'-CGATCATTTCTT GTACTGCTCTC-3' | |
Sequence-based reagent | CrLFY1invF3 | This paper | Inverse PCR, CrLFY1 : 5'-CAGTGCATGACCTTCGATATTG-3' | |
Sequence-based reagent | CrLFY1invR | This paper | Inverse PCR, CrLFY1: 5'-CAGTTGTTTCGGATCTGCAG-3' | |
Sequence-based reagent | CrLFY1invR2 | This paper | Inverse PCR, CrLFY1: 5'-CTCCGCTTTTCATTTGAGAACG-3' | |
Sequence-based reagent | CrLFY1invR3 | This paper | Inverse PCR, CrLFY1: 5'-CAAGAACCGCTGGAGTAAAC-3' | |
Sequence-based reagent | CrLFY2invF | This paper | Inverse PCR, CrLFY2: 5'-CTATGGTGTACGGAGCACTAC-3' | |
Sequence-based reagent | CrLFY2invF2 | This paper | Inverse PCR, CrLFY2: 5'-CGTATCCAAAACAGC TTAAACTCC-3' | |
Sequence-based reagent | CrLFY2invF3 | This paper | Inverse PCR, CrLFY2: 5'-CACTAAAGGTGCTGCTATCAAC-3' | |
Sequence-based reagent | CrLFY2invF4 | This paper | Inverse PCR, CrLFY2: 5'-CATTGTGCTGACCTTGTGAAG-3' | |
Sequence-based reagent | CrLFY2invF5 | This paper | Inverse PCR, CrLFY2: 5'-CGCAAAGGTTGGAA AAGAGAAC-3' | |
Sequence-based reagent | CrLFY2invF6 | This paper | Inverse PCR, CrLFY2: 5'-CGACAACGGATCATAACCATC-3' | |
Sequence-based reagent | CrLFY2 invF7 | This paper | Inverse PCR, CrLFY2: 5'-CAATAGTAGATT CTCCCTCCTTTAC-3' | |
Sequence-based reagent | CrLFY2invF8 | This paper | Inverse PCR, CrLFY2: 5'-GCTCTTTAATTT GAATCACGTGTG-3' | |
Sequence-based reagent | CrLFY2invF9 | This paper | Inverse PCR, CrLFY2: 5'-GAACAATGTGCA TGCGACTC-3' | |
Sequence-based reagent | CrLFY2invF10 | This paper | Inverse PCR, CrLFY2: 5'-CATGTTCCGAT GGGAACAAAG-3' | |
Sequence-based reagent | CrLFY2invF11 | This paper | Inverse PCR, CrLFY2: 5'-CATAGGGAACT CTGTAATGATGC-3' | |
Sequence-based reagent | CrLFY2invF12 | This paper | Inverse PCR, CrLFY2: 5'-GTTTCCAG ATACTGCTGCTC-3' | |
Sequence-based reagent | CrLFY2invF13 | This paper | Inverse PCR, CrLFY2: 5'-CATAGATGA TGCCAGTATACTCC-3' | |
Sequence-based reagent | CrLFY2invF14 | This paper | Inverse PCR, CrLFY2: 5'-GCTCACTAT CCACAATTCATACAC-3' | |
Sequence-based reagent | CrLFY2invF15 | This paper | Inverse PCR, CrLFY2: 5'-GTTCGTATCT GATACTTGTTTCGTG-3' | |
Sequence-based reagent | CrLFY2invF16 | This paper | Inverse PCR, CrLFY2: 5'-CTTACTCCA CGAATGCATGC-3' | |
Sequence-based reagent | CrLFY2invR | This paper | Inverse PCR, CrLFY2: 5'-CAGTTGTCAC AGAGGTAGCAG-3' | |
Sequence-based reagent | CrLFY2invR2 | This paper | Inverse PCR, CrLFY2: 5'-CCTTACGATG TATTACCCTTTGTTC-3' | |
Sequence-based reagent | CrLFY2invR3 | This paper | Inverse PCR, CrLFY2: 5'-CAGTGACTA GGATGTCTGATACAG-3' | |
Sequence-based reagent | CrLFY2invR4 | This paper | Inverse PCR, CrLFY2: 5'-GAAGGAGCT GAAAATGCAACTC-3' | |
Sequence-based reagent | CrLFY2invR5 | This paper | Inverse PCR, CrLFY2: 5'-CCTGCCTCC TATGAAAACAC-3' | |
Sequence-based reagent | CrLFY2invR6 | This paper | Inverse PCR, CrLFY2: 5'-CCTGTAAAGG AGGGAGAATCTAC-3' | |
Sequence-based reagent | CrLFY2invR7 | This paper | Inverse PCR, CrLFY2: 5'-GCACTCCAAC GATGATGATAC-3' | |
Sequence-based reagent | CrLFY2invR8 | This paper | Inverse PCR, CrLFY2: 5'-GCTGTACTA AGGCATCAATTCAG-3' | |
Sequence-based reagent | CrLFY2invR9 | This paper | Inverse PCR, CrLFY2: 5'-CATCTATGATA GCACAACATCACTC-3' | |
Sequence-based reagent | CrLFY2invR10 | This paper | Inverse PCR, CrLFY2: 5'-CACAACATC ACTCAGGACTC-3' | |
Sequence-based reagent | CrLFY2invR11 | This paper | Inverse PCR, CrLFY2: 5'-CTGCCTCCTA TGAAAACACAAG-3' | |
Sequence-based reagent | CrLFY2invR12 | This paper | Inverse PCR, CrLFY2: 5'-CTAGTCTTTG ATGAGGTTTCATGTC-3' | |
Sequence-based reagent | CrLFY2invR13 | This paper | Inverse PCR, CrLFY2: 5'-CATGCAAGA AGCATGCAATTC-3' | |
Sequence-based reagent | CrLFY2invR14 | This paper | Inverse PCR, CrLFY2: 5'-GTGTCTCCA GTAAGTATGAAACAAG-3' | |
Sequence-based reagent | CrLFY2invR15 | This paper | Inverse PCR, CrLFY2: 5'-CATGAGGCC GTCAGACTTAC-3' | |
Sequence-based reagent | CrLFY2invR16 | This paper | Inverse PCR, CrLFY2: 5'-CGTAACAGA CGAGCTCGATATAATAG-3' | |
Sequence-based reagent | CrLFY2invR17 | This paper | Inverse PCR, CrLFY2: 5'-CTCTTTGCTCA TATAGCTTCAAGC-3' | |
Sequence-based reagent | CrLFY1 + 2 (1)-RNAi-F | This paper | T-DNA cloning, CrLFY1/2-i1: 5'-ATGGGT TTCACTGTGAATAC-3' | |
Sequence-based reagent | CrLFY1 + 2 (1)-RNAi-R | This paper | T-DNA cloning, CrLFY1/2-i1: 5'-TCTCCTC TTTGTTCCCTTGTG-3' | |
Sequence-based reagent | CrLFY1 + 2 (2)-RNAi-F | This paper | T-DNA cloning, CrLFY1/2-i2: 5'-ATGGG TTTCACTGTTAGTAC-3' | |
Sequence-based reagent | CrLFY1 + 2 (2)-RNAi-R | This paper | T-DNA cloning, CrLFY1/2-i2: 5'-TCTCCT CTTTGTTCCCTGGTG-3' | |
Sequence-based reagent | CrLFY1-RNAi-F | This paper | T-DNA cloning, CrLFY1-i3: 5'-CCTTTTCT TGCTAATGATGGC-3' | |
Sequence-based reagent | CrLFY1-RNAi-R | This paper | T-DNA cloning, CrLFY1-i3: 5'-CAAACAAA CTTGAAAATGATAC-3' | |
Sequence-based reagent | CrLFY2-RNAi-F | This paper | T-DNA cloning, CrLFY2-i4: 5'-GCCATTG CTAGCAAGGTTAT-3' | |
Sequence-based reagent | CrLFY2-RNAi-R | This paper | T-DNA cloning, CrLFY2-i4: 5'-CACTGCT TTGAAACTAAAAC-3' | |
Sequence-based reagent | pCrLFY1amp-NotF | This paper | T-DNA cloning, CrLFY1pro: 5'-CAGCGGCCGCTTAGATGG CTTGAGATGCTAC-3' | |
Sequence-based reagent | pCrLFY1amp-XbaR | This paper | T-DNA cloning, CrLFY1pro: 5'-CATCTAGAG GAGGCACTTCTTTACGTG-3' | |
Sequence-based reagent | GUSamp-XbaF | This paper | T-DNA cloning, GUS CDS: 5'-CATCTAGAC AATGGTAAGCTTAGCGGG-3' | |
Sequence-based reagent | GUSamp-XbaR | This paper | T-DNA cloning, GUS CDS: 5'-CCATCTAGA TTCATTGTTTGCCTCCCTG-3' | |
Sequence-based reagent | qCrLFY1_F2 | This paper | qRT-PCR, CrLFY1: 5'-GTCCGCT ATTCGTGCAGAGA-3' | |
Sequence-based reagent | qCrLFY1_R2 | This paper | qRT-PCR, CrLFY1 : 5'-AATTCAAGGGGG CATTGGGT-3' | |
Sequence-based reagent | qCrLFY2_F3 | This paper | qRT-PCR, CrLFY2: 5'-GCAGTGACAATGAAGGACGC-3' | |
Sequence-based reagent | qCrLFY2_R3 | This paper | qRT-PCR, CrLFY2: 5'-AGAATCGTGCACACTGCTCA-3' | |
Sequence-based reagent | qCrTBPb_F | Ganger et al. (2015), DOI:10.1139/cjb-2014–0202 | qRT-PCR, CrTBP: 5'-ATGAGCCAGAGCTTTTCCCC-3' | |
Sequence-based reagent | qCrTBPb_R | Ganger et al. (2015), DOI:10.1139/cjb-2014–0202 | qRT-PCR, CrTBP: 5'-TTCGTCTCTGACCTTTGCCC-3' | |
Sequence-based reagent | qCrACT1_F | Ganger et al. (2015), DOI:10.1139/cjb-2014–0202 | qRT-PCR, CrActin1: 5'-GAGAGAGGCTA CTCTTTCACAACC-3' | |
Sequence-based reagent | qCrACT1_R | Ganger et al. (2015), DOI:10.1139/cjb-2014–0202 | qRT-PCR, CrActin1: 5'-AGGAAGTTCGTA ACTCTTCTCCAA-3' | |
Sequence-based reagent | CrLFY1_ISH_F | This paper | In situ probes, CrLFY1: 5'-GAGGCATACA CACACGCAGT-3' | |
Sequence-based reagent | CrLFY1_ISH_R | This paper | In situ probes, CrLFY1: 5'-TCAATCATAGAT GCAGCTATCACTG-3 | |
Sequence-based reagent | CrLFY2_ISH_F | This paper | In situ probes, CrLFY2: 5'-GGCTGGTTGTTA CGGATAGC-3' | |
Sequence-based reagent | CrLFY2_ISH_R | This paper | In situ probes, CrLFY2: 5'-TTATTCATAG CTGCAGCTGTCACTG-3' | |
Sequence-based reagent | CrLFY1_Probe1F | This paper | Copy number analysis, CrLFY1 probe 1: 5'-CAGG CACAAGGGAACAAAG-3' | |
Sequence-based reagent | CrLFY1_Probe1R | This paper | Copy number analysis, CrLFY1 probe 1: 5'-CA TAGATGCAGCTATCACTGTC-3' | |
Sequence-based reagent | CrLFY1_Probe2F | This paper | Copy number analysis, CrLFY1 probe 2: 5'-CACTTGAAGGTAAGCT TTATTGTAAGG-3' | |
Sequence-based reagent | CrLFY1_Probe2R | This paper | Copy number analysis, CrLFY1 probe 2: 5'-CAATA TTTCCGACTATACATTGAGGC-3' | |
Sequence-based reagent | CrLFY2_Probe1F | This paper | Copy number analysis, CrLFY2 probe 1: 5'-CAGGCA CCAGGGAACAAAG-3' | |
Sequence-based reagent | CrLFY2_Probe1R | This paper | Copy number analysis, CrLFY2 probe 1: 5'-CATAGC TGCAGCTGGTCACTGTC-3' | |
Sequence-based reagent | CrLFY2_Probe2F | This paper | Copy number analysis, CrLFY2 probe 2: 5'-CTGTAG AAGGTAAGATTCTGCTC-3' | |
Sequence-based reagent | CrLFY2_Probe2R | This paper | Copy number analysis, CrLFY2 probe 2: 5'-GCTT ATGGTACAGAATAAGTAGAGG-3' | |
Sequence-based reagent | HygF2 | Plackett et al. (2014), PMID:24623851 | T-DNA gel blot probe, HygR: 5'-CTTCTACA CAGCCATCGGTC-3' | |
Sequence-based reagent | HygR | Plackett et al. (2014), PMID:24623851 | T-DNA gel blot probe, HygR: 5'-CCGATGGT TTCTACAAAGATCG-3' | |
Sequence-based reagent | GH3seqF3 | Plackett et al. (2014), PMID:24623851 | T-DNA gel blot probe, GUS: 5'-CTTCGCT GTACAGTTCTTTCG-3' | |
Sequence-based reagent | GH3seqR4 | Plackett et al. (2014), PMID:24623851 | T-DNA gel blot probe, GUS: 5'-CACTCATT ACGGCAAAGTGTG-3' | |
Sequence-based reagent | GUSlinkerseqF | This paper | T-DNA gel blot probe, RNAi: 5'-CTGATT AACCACAAACCGTTCTAC-3' | |
Sequence-based reagent | GUSlinkerseqR | This paper | T-DNA gel blot probe, RNAi: 5'-CTGATA CTCTTCACTCCACATG-3' | |
Sequence-based reagent | HPT-F | Miki and Shimamoto (2004), PMID:15111724 | RNAi genotyping, HygR: 5'-GAGCCTGACCTA TTGCATCTCC-3' | |
Sequence-based reagent | HPT-R | Miki and Shimamoto (2004), PMID:15111724 | RNAi genotyping, HygR: 5'-GGCCTCCAG AAGAAGATGTTGG-3' | |
Sequence-based reagent | pVec8F | Miki and Shimamoto (2004), PMID:15111724 | RNAi genotyping, RNAi hairpin: 5'-TTTAGC CCTGCCTTCATACG-3' | |
Sequence-based reagent | pVec8R | Miki and Shimamoto (2004), PMID:15111724 | RNAi genotyping, RNAi hairpin: 5'-ATTGC CAAATGTTTGAACGA-3' | |
Sequence-based reagent | PW64F | This paper | RNAi genotyping, RNAi hairpin: 5'-CATGAA GATGCGGACTTACG-3' | |
Sequence-based reagent | PW64R | This paper | RNAi genotyping, RNAi hairpin: 5'-ATCCAC GCCGTATTCGG-3' | |
Sequence-based reagent | pCrLFY1genoF1 | This paper | CrLFY1pro::GUS genotyping: 5'-CTTAGA TGGCTTGAGATGCTAC-3' | |
Sequence-based reagent | pCrLFY1genoF2 | This paper | CrLFY1pro::GUS genotyping: 5'-CTCTCT TCTTGCTTGTGTTGTG-3' | |
Sequence-based reagent | pCrLFY1genoF3 | This paper | CrLFY1pro::GUS genotyping: 5'-CAACTGGCAACAGGTGATG-3' | |
Sequence-based reagent | pCrLFY1genoF4 | This paper | CrLFY1pro::GUS genotyping: 5'-CAGTCTTAGTTCAACTGCATTCG-3' | |
Sequence-based reagent | pCrLFY1genoR | This paper | CrLFY1pro::GUS genotyping: 5'-AGGAGGCACTTCTTTACGTG-3' | |
Sequence-based reagent | GUSgenoR | This paper | CrLFY1pro::GUS + 35Spro::GUS genotyping: 5'-CATTGTTTG CCTCCCTGC-3' | |
Sequence-based reagent | 35SgenoF | This paper | 35Spro::GUS genotyping: 5'-CTGAGCTTAACAGCACAGTTG-3' | |
Sequence-based reagent | OCS3’genoR | This paper | 35Spro::GUS genotyping: 5'-CATCACTAGTAAGCTAGCTTGC-3' | |
Commercial assay or kit | Phusion high-fidelity polymerase | Thermo Scientific | Thermo Scientific: F530S | |
Commercial assay or kit | Gateway LR clonase II enzyme mix | Invitrogen | Thermo Scientific: 11791100 | |
Commercial assay or kit | QIAGEN Plasmid Maxi Kit | QIAGEN | QIAGEN:12163 | |
Commercial assay or kit | Whatman Nytran nylon blotting membrane | GE Healthcare | GE Healthcare: 10416294 | |
Commercial assay or kit | Random Primers DNA Labelling kit | Invitrogen | Thermo Scientific: 18187013 | |
Commercial assay or kit | Carestream Kodak autoradiography GBX developer and fixer | Sigma-Aldrich | Sigma-Aldrich: Z354147 | |
Commercial assay or kit | Carestream Kodak Biomax XAR film | Sigma-Aldrich | Sigma-Aldrich:F5763 | |
Commercial assay or kit | iTaq universal SYBR Green mastermix | Bio-Rad | Bio-Rad:1725120 | |
Commercial assay or kit | DIG-labelling mix | Roche Applied Sciences | Roche:11277073910 | |
Commercial assay or kit | T3 RNA polymerase | Roche Applied Sciences | Roche:11031163001 | |
Commercial assay or kit | T7 RNA polymerase | Roche Applied Sciences | Roche:10881767001 | |
Commercial assay or kit | Anti-DIG antibody | Roche Applied Sciences | Roche:11093274910; RRID:AB_2313639 | |
Commercial assay or kit | NBT/BCIP stock solution | Roche Applied Sciences | Roche:11681451001 | |
Chemical compound, drug | Potassium ferricyanide (K₃Fe(CN)₆) | Sigma-Aldrich | Sigma:P8131 | |
Chemical compound, drug | X-GlcA (CHA salt) | Melford Scientific | Melford:MB1021 | |
Chemical compound, drug | CTP, [ɑ-32P] | Perkin Elmer | Perkin Elmer: BLU008H250UC | |
Software, algorithm | IQ-TREE | Nguyen et al. (2015), PMID:25371430 | http://www.iqtree.org/ | |
Software, algorithm | iTOL | Letunic and Bork (2016), PMID:27095192 | https://itol.embl.de/ | |
Software, algorithm | ClustalW | Li et al. (2015), PMID:25845596 | RRID:SCR_002909 | https://www.ebi.ac.uk/Tools/msa/clustalw2/ |
Software, algorithm | TBLASTX | Altschul et al. (1990), PMID:2231712 | RRID:SCR_011823 | https://blast.ncbi.nlm.nih.gov/Blast.cgi |
Software, algorithm | GeneWise | Birney et al. (2004), PMID:15123596 | RRID:SCR_015054 | https://www.ebi.ac.uk/Tools/psa/genewise |
Software, algorithm | GraphPad Prism | GraphPad Software Inc. | RRID:SCR_002798 | https://www.graphpad.com/scientific-software/prism/ |
Software, algorithm | Adobe Photoshop CS4 | Adobe | RRID:SCR_014199 | |
Other | Biolistic PDS-1000/He Particle Delivery System | Bio-Rad | Bio-Rad:1652257 | |
Other | CFX Connect Real-Time PCR Detection System | Bio-Rad | Bio-Rad:1855201 | |
Other | Zeiss Axioplan microscope | Zeiss | ||
Other | Nikon Microphot-FX microscope | Nikon | ||
Other | MicroPublisher 3.3 RTV camera | Qimaging |
Additional files
-
Supplementary file 1
LFY sequences included in phylogenetic analysis (in addition to Sayou et al., 2014) dataset).
- https://doi.org/10.7554/eLife.39625.027
-
Supplementary file 2
Alignment of all 120 LFY amino acid sequences used in phylogenetic analysis.
- https://doi.org/10.7554/eLife.39625.028
-
Supplementary file 3
Alignment of LFY amino acid sequences used in phylogenetic analysis (ferns only).
- https://doi.org/10.7554/eLife.39625.029
-
Supplementary file 4
Statistical comparison of CrLFY transcript levels between different ontogenetic stages.
- https://doi.org/10.7554/eLife.39625.030
-
Supplementary file 5
Specificity of CrLFY RNAi target sequences.
Alignment (prepared using Clustal Omega) of the full length transcript sequences for CrLFY1 and CrLFY2, with nucleotide identity between the two gene copies denoted by a subtending asterisk. The CDS for each gene is highlighted in bold. The target sequences for each RNAi construct are highlighted in light blue (CrLFY1/2-i1), light green (CrLFY1/2-i2), dark blue (CrLFY1-i3) or dark green (CrLFY2-i4). The CrLFY1/2-i1 and CrLFY1/2-i2 target sequences each have 77% similarity to the opposing gene transcript (BLAST2n, discontiguous megablast for highly similar sequences). The full-length CrLFY1-i3 and CrLFY2-i4 target sequences do not demonstrate significant sequence similarity to the opposing gene transcript or 3’UTR alone (BLAST2n, blastn for somewhat similar sequences) but short regions of similarity within the target sequence might explain the cross-reactivity observed.
- https://doi.org/10.7554/eLife.39625.031
-
Supplementary file 6
Predicted hybridization and specificity of CrLFY in situ hybridization probes.
Alignment (prepared using Clustal Omega) of full length CrLFY1 and CrLFY2 transcript sequences, with nucleotide identity between the two paralogs denoted by a subtending asterisk. The coding sequence (CDS) for each gene copy is highlighted in bold. Predicted sites of hybridization for the two probes are highlighted in blue (CrLFY1) and yellow (CrLFY2) respectively, with PCR primer sites underlined. The in situ probes span the complete CDS and 5’UTR of each gene copy. The CrLFY1 probe sequence shows 79% nucleotide identity to the CrLFY2 transcript (BLAST2n, discontiguous megablast for highly similar sequences). The CrLFY2 probe shows 79% nucleotide identity to the CrLFY1 transcript (BLAST2n, discontiguous megablast for highly similar sequences).
- https://doi.org/10.7554/eLife.39625.032
-
Supplementary file 7
Amplified CrLFY2 genomic fragment (3619 bp), not connected directly to CrLFY2 open reading frame.
Sequence highlighted in green corresponds to published CrLFY2 5’UTR (Himi et al., 2001)
- https://doi.org/10.7554/eLife.39625.033
-
Supplementary file 8
Summary of published reports of LFY function in a range of angiosperm species.
All citations included in reference list of main article (Bradley et al., 1996; Blázquez et al., 1997; Bradley et al., 1997; Kyozuka et al., 1998; Pnueli et al., 1998; Ratcliffe et al., 1999; Gourlay et al., 2000; Bomblies et al., 2003; Becker et al., 2005; Meng et al., 2007; Souer et al., 2008; Wreath et al., 2013).
- https://doi.org/10.7554/eLife.39625.034
-
Transparent reporting form
- https://doi.org/10.7554/eLife.39625.035