Evolution: Of starch and spit
Rice, bread and potatoes are just a few of the starchy foods that many human populations rely on in their day-to-day lives. In fact, starches have probably been part of our diet for a long time. Starch residues have been found in cooking hearths from 120,000 years ago, when the Sahara desert was still covered in lush vegetation, and cereals were some of the first crops to be domesticated and farmed (Larbey et al., 2019; Simmons, 2011). This close relationship with starch has inevitably left its mark on our genes.
Starch is formed of many sugar molecules attached together, so it must be cut into smaller pieces to be absorbed into our bloodstream: the cutting up process is guided by an enzyme called alpha-amylase. This protein is encoded by the AMY gene family, and in a few species such as humans, it is expressed in both the pancreas and saliva.
Modern humans differ from other primates, and even other species of early humans, in how many copies of the AMY genes we have. Unlike Neanderthals and Denisovans, who had only two diploid copies, we carry up to 20 copies of the AMY1 gene, which produces salivary amylase. In fact, there is evidence that this gene was strongly selected for as early as the middle Pleistocene, when modern humans emerged (Inchley et al., 2016). This may suggest that our lineage has evolved specific adaptations to digest starch-rich foods, underlining the long and continuing importance of these staples in our diet (Hardy et al., 2015).
Several studies have found that the higher the number of AMY1 gene copies, the more amylase protein is expressed in saliva (Perry et al., 2007; Carpenter et al., 2017). This may affect how well we digest starchy foods, but also how we may perceive them. Indeed, as the amylase in saliva breaks down starch in the mouth, it releases a sweet taste that we can detect (Mandel et al., 2010; Mandel and Breslin, 2012).
Of course, humans vary in how much starch they consume; strikingly, populations that have higher numbers of amylase gene copies and more amylase in their saliva also tend to eat more starch (Perry et al., 2007; Inchley et al., 2016). A similar pattern is found in wolves and their domesticated counterpart, the dog. While wolves only have two diploid copies of the amylase gene, dogs can have many, and they also have higher levels of amylase expression and activity in their pancreas (Axelsson et al., 2013). As wolves became dogs, their behavior and appearance changed, but they also adapted to a new source of food that was rich in starch: human food scraps (Figure 1A,B).
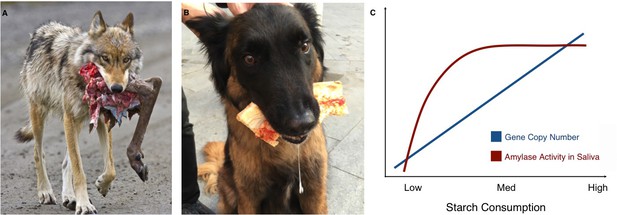
Links between proximity to humans, amylase gene copy number and amylase activity.
Animals that live alongside humans have diets that are different to those of their wild relatives, and these differences have led to dietary adaptations. While wolves (A) are highly carnivorous, dogs (B) have adapted to eating starchy human food scraps. (C) Pajic et al. found that species that consume medium or high amounts of starch have higher amylase activity in their saliva (maroon line) and more amylase gene copies (blue line) than species that consume little or no starch.
Image credit: A. NPS Photo/Ken Conger (CC BY 2.0); B. Mareike Janiak (CC BY 4.0)
Now, in eLife, Stefan Ruhl and Omer Gokcumen of the University at Buffalo and colleagues, including Petar Pajic as first author, report that dogs are not the only species living alongside humans that have adapted to eating more starch (Pajic et al., 2019). The team collected data on amylase gene copy numbers and salivary amylase activity from a range of different mammals, many of which had never been measured before. For several species, both the wild and domesticated (or at least commensal) varieties were sampled, such as wolves and dogs, wild and house (or street) mice and rats, and boars and pigs.
The analyses show that species living in close quarters with humans have more copies of the amylase genes than their wild relatives, as well as higher levels of amylase activity in their saliva. This is even the case for species whose ‘natural’ diets already contained a fair amount of starch, such as mice. Compared to wild deer mice, house mice have even more amylase genes and activity, possibly because of the many starchy foods we (unwittingly) provide.
The results also offer new insights into the ways copy number variations might affect the activity of amylase in saliva. While the number of amylase genes increases almost linearly with starch consumption, there is no difference in amylase activity in the saliva of species with intermediate or high starch consumption. Both have much more amylase in their saliva than species with very low or no starch intake, but in species with high starch consumption, the additional copies of the amylase gene do not seem to translate to higher amounts of salivary enzyme (Figure 1C). Similarly, some species with very high amylase activity in their saliva, such as baboons or macaques, do not have a corresponding increase in amylase gene copies. These unexpected findings should encourage research into the other mechanisms that may affect the activity of salivary amylase. Another avenue of study could be to look at the pancreatic activity of the enzyme. Pajic et al. – who are based in Greece, Germany and several institutes in the US – only measured salivary amylase, but the enzyme is also produced in the pancreas: for example, this is where dogs translate their many amylase gene copies into amylase activity (Axelsson et al., 2013).
Humans have adapted to efficiently digest new foods such as milk and grains (Janiak, 2016), but our dietary habits and our love for starchy carbohydrates might also have shaped the animals that live amongst us. The new findings by Pajic et al. therefore illustrate how powerful our behaviors are at influencing our environment.
References
-
The importance of dietary carbohydrate in human evolutionThe Quarterly Review of Biology 90:251–268.https://doi.org/10.1086/682587
-
Digestive enzymes of human and nonhuman primatesEvolutionary Anthropology: Issues, News, and Reviews 25:253–266.https://doi.org/10.1002/evan.21498
-
Cooked starchy food in hearths ca. 120 kya and 65 kya (MIS 5e and MIS 4) from Klasies River Cave, South AfricaJournal of Human Evolution 131:210–227.https://doi.org/10.1016/j.jhevol.2019.03.015
-
Diet and the evolution of human amylase gene copy number variationNature Genetics 39:1256–1260.https://doi.org/10.1038/ng2123
-
BookThe Neolithic Revolution in the Near East: Transforming the Human LandscapeTucson, AZ: University of Arizona Press.
Article and author information
Author details
Publication history
Copyright
© 2019, Janiak
This article is distributed under the terms of the Creative Commons Attribution License, which permits unrestricted use and redistribution provided that the original author and source are credited.
Metrics
-
- 15,312
- views
-
- 328
- downloads
-
- 3
- citations
Views, downloads and citations are aggregated across all versions of this paper published by eLife.
Download links
Downloads (link to download the article as PDF)
Open citations (links to open the citations from this article in various online reference manager services)
Cite this article (links to download the citations from this article in formats compatible with various reference manager tools)
Further reading
-
- Evolutionary Biology
With the availability of high-quality full genome polymorphism (SNPs) data, it becomes feasible to study the past demographic and selective history of populations in exquisite detail. However, such inferences still suffer from a lack of statistical resolution for recent, for example bottlenecks, events, and/or for populations with small nucleotide diversity. Additional heritable (epi)genetic markers, such as indels, transposable elements, microsatellites, or cytosine methylation, may provide further, yet untapped, information on the recent past population history. We extend the Sequential Markovian Coalescent (SMC) framework to jointly use SNPs and other hyper-mutable markers. We are able to (1) improve the accuracy of demographic inference in recent times, (2) uncover past demographic events hidden to SNP-based inference methods, and (3) infer the hyper-mutable marker mutation rates under a finite site model. As a proof of principle, we focus on demographic inference in Arabidopsis thaliana using DNA methylation diversity data from 10 European natural accessions. We demonstrate that segregating single methylated polymorphisms (SMPs) satisfy the modeling assumptions of the SMC framework, while differentially methylated regions (DMRs) are not suitable as their length exceeds that of the genomic distance between two recombination events. Combining SNPs and SMPs while accounting for site- and region-level epimutation processes, we provide new estimates of the glacial age bottleneck and post-glacial population expansion of the European A. thaliana population. Our SMC framework readily accounts for a wide range of heritable genomic markers, thus paving the way for next-generation inference of evolutionary history by combining information from several genetic and epigenetic markers.
-
- Computational and Systems Biology
- Evolutionary Biology
There is growing interest in designing multidrug therapies that leverage tradeoffs to combat resistance. Tradeoffs are common in evolution and occur when, for example, resistance to one drug results in sensitivity to another. Major questions remain about the extent to which tradeoffs are reliable, specifically, whether the mutants that provide resistance to a given drug all suffer similar tradeoffs. This question is difficult because the drug-resistant mutants observed in the clinic, and even those evolved in controlled laboratory settings, are often biased towards those that provide large fitness benefits. Thus, the mutations (and mechanisms) that provide drug resistance may be more diverse than current data suggests. Here, we perform evolution experiments utilizing lineage-tracking to capture a fuller spectrum of mutations that give yeast cells a fitness advantage in fluconazole, a common antifungal drug. We then quantify fitness tradeoffs for each of 774 evolved mutants across 12 environments, finding these mutants group into classes with characteristically different tradeoffs. Their unique tradeoffs may imply that each group of mutants affects fitness through different underlying mechanisms. Some of the groupings we find are surprising. For example, we find some mutants that resist single drugs do not resist their combination, while others do. And some mutants to the same gene have different tradeoffs than others. These findings, on one hand, demonstrate the difficulty in relying on consistent or intuitive tradeoffs when designing multidrug treatments. On the other hand, by demonstrating that hundreds of adaptive mutations can be reduced to a few groups with characteristic tradeoffs, our findings may yet empower multidrug strategies that leverage tradeoffs to combat resistance. More generally speaking, by grouping mutants that likely affect fitness through similar underlying mechanisms, our work guides efforts to map the phenotypic effects of mutation.