Alzheimer’s Disease: Exploring the origins of nucleation
Alzheimer’s disease is a progressive neurodegenerative disease that initially compromises memory and ultimately stops people from being able to perform everyday tasks. The exact causes of the disease are still unknown, but genetic, lifestyle and environmental factors could all play a role, with age being the strongest risk factor for developing the condition. The lack of treatment to halt or slow down the progression Alzheimer’s disease makes it one of the greatest challenges of our times.
Alzheimer’s disease begins decades before the appearance of clinical symptoms. It is thought that changes in the metabolism of a peptide called amyloid beta (Aβ) lead to its misfolding. Although the details are not fully understood, scientists propose that the accumulation of these misfolded molecules in the brain triggers a series of molecular events which, in turn, lead to neuroinflammation, the aggregation of tau proteins in neurons, and eventually, neuronal death (Selkoe and Hardy, 2016).
Preventing the build-up of Aβ has been explored as a potential therapeutic approach. However, designing strategies that specifically target the formation of neurotoxic Aβ, rather than the aggregation of Aβ in general, requires a detailed understanding (in terms of both mechanisms and kinetics) of how the protein misfolds and how this is connected to the disease (Yang et al., 2017; Brinkmalm et al., 2019). Now, in eLife, Benedetta Bolognesi (IBEC in Barcelona), Ben Lehner (the Center for Genomic Regulation, also in Barcelona) and colleagues – including Mireia Seuma as first author, Andre Faure and Marta Badia – report new insights into how mutations affect the initial nucleation of Aβ aggregates (Seuma et al., 2021).
The researchers used an approach called deep mutational scanning (Gray et al., 2019) to generate a library of 468 single and 14,015 double mutant Aβ variants to see how the mutations affect the ability of Aβ to form new aggregates. They focused on the first step of this process, known as nucleation, and then determined the relationship between amino acid sequence and the nucleation rate of Aβ42 (a form of Aβ that contains 42 amino acids) (Figure 1).
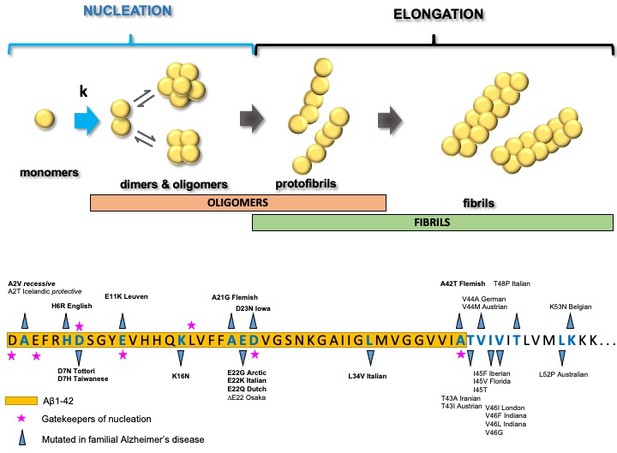
Amyloid beta peptide and Alzheimer’s disease.
It is thought that Alzheimer’s disease is caused by amyloid beta (Aβ) (yellow circles; top) first forming dimers and then oligomers in a process called nucleation, with the oligomers then going on to build protofibrils and fibrils. Aggregated Aβ then deposits in amyloid plaques, which are a pathological hallmark of Alzheimer’s disease. Familial Alzheimer’s disease is hereditary and is marked by an unusually early onset of symptoms. Several genes have been linked to this form of the disease, including the gene for the amyloid precursor protein (APP). Certain amino acid residues in Aβ are called 'gatekeepers’ of nucleation (magenta stars; bottom) because they prevent nucleation. The mutations indicated (blue arrows), affecting amino acid residues highlighted in blue, cause familial Alzheimer’s disease or protect (A2T) against it. Pathogenic mutations in APP are shown in text (the numbering corresponds to the positions with Aβ). The sequence of amino acids shown here is from the N-terminal of Aβ42. Seuma et al. found that dominant pathogenic mutations within the Aβ42 peptide (indicated in bold text; bottom) all display increased rate of nucleation.
Seuma et al. found that mutations that reduce nucleation are clustered in the hydrophobic part of Aβ42 (the C-terminal), while those that increase this process are located in the N-terminal part, which is hydrophilic. This suggests that Aβ is organized in a modular manner, with the N- and C-terminal parts having different roles in nucleation and aggregation. Intriguingly, such a modularity is also reflected in the distribution of pathogenic mutations in the Aβ precursor called APP (Figure 1). These mutations have been linked to familial Alzheimer’s disease, a rare genetic form presenting a much earlier onset (people usually develop symptoms in their thirties, forties and fifties).
Seuma et al. further identified five negatively charged residues in Aβ42, which acted as 'gatekeepers’, preventing the peptides from sticking to each other (Rousseau et al., 2006). Mutations at these sites frequently resulted in increased nucleation. This raises several questions: could the gatekeepers of nucleation prevent neurodegeneration; and do mutations that promote nucleation prime the system for the onset of Alzheimer’s disease?
To investigate this further, Seuma et al. studied the nucleation rates for 12 mutations in Aβ42, which have been linked to familial Alzheimer’s disease. The mutations all resulted in increased nucleation rates, although the rates did not correlate with the severity of the disease (as reflected by the age at disease onset). These results suggest that Aβ nucleation plays a key role in the formation of neurotoxic aggregates, but other factors are also involved.
It is worth noting that the reservoir of Aβ in the brain contains a heterogeneous mixture of peptides of varying lengths, generated by the sequential cleavage of APP. Pathogenic mutations in both APP and the enzymes responsible for its cleavage favor the production of longer Aβ peptides (Szaruga et al., 2017). Importantly a large number of mutations linked to familial Alzheimer’s disease do not change the amino acid sequence of Aβ42, but affect the composition of Aβ profiles by shifting them towards longer peptides (Szaruga et al., 2015). Whether these pathogenic changes in peptide length (and hydrophobicity) lead to an increase in nucleation, and how this is related to the clinical phenotypes of Alzheimer’s disease, warrant further investigation.
Disentangling the complex pathogenesis of Alzheimer’s disease will likely require the interrogation of various pathogenic variants under controlled conditions and the plotting of biochemical and cellular data against clinical severity of the condition. The study by Seuma et al. certainly shows that the nucleation assay is a valuable tool for such investigations.
References
-
Elucidating the molecular determinants of aβ aggregation with deep mutational scanningG3: Genes, Genomes, Genetics 9:3683–3689.https://doi.org/10.1534/g3.119.400535
-
How evolutionary pressure against protein aggregation shaped chaperone specificityJournal of Molecular Biology 355:1037–1047.https://doi.org/10.1016/j.jmb.2005.11.035
-
The amyloid hypothesis of Alzheimer's disease at 25 yearsEMBO Molecular Medicine 8:595–608.https://doi.org/10.15252/emmm.201606210
-
Qualitative changes in human γ-secretase underlie familial Alzheimer’s diseaseJournal of Experimental Medicine 212:2003–2013.https://doi.org/10.1084/jem.20150892
Article and author information
Author details
Publication history
Copyright
© 2021, Zoltowska and Chávez-Gutiérrez
This article is distributed under the terms of the Creative Commons Attribution License, which permits unrestricted use and redistribution provided that the original author and source are credited.
Metrics
-
- 1,293
- views
-
- 130
- downloads
-
- 0
- citations
Views, downloads and citations are aggregated across all versions of this paper published by eLife.
Download links
Downloads (link to download the article as PDF)
Open citations (links to open the citations from this article in various online reference manager services)
Cite this article (links to download the citations from this article in formats compatible with various reference manager tools)
Further reading
-
- Computational and Systems Biology
The RAS-MAPK system plays an important role in regulating various cellular processes, including growth, differentiation, apoptosis, and transformation. Dysregulation of this system has been implicated in genetic diseases and cancers affecting diverse tissues. To better understand the regulation of this system, we employed information flow analysis based on transfer entropy (TE) between the activation dynamics of two key elements in cells stimulated with EGF: SOS, a guanine nucleotide exchanger for the small GTPase RAS, and RAF, a RAS effector serine/threonine kinase. TE analysis allows for model-free assessment of the timing, direction, and strength of the information flow regulating the system response. We detected significant amounts of TE in both directions between SOS and RAF, indicating feedback regulation. Importantly, the amount of TE did not simply follow the input dose or the intensity of the causal reaction, demonstrating the uniqueness of TE. TE analysis proposed regulatory networks containing multiple tracks and feedback loops and revealed temporal switching in the reaction pathway primarily responsible for reaction control. This proposal was confirmed by the effects of an MEK inhibitor on TE. Furthermore, TE analysis identified the functional disorder of a SOS mutation associated with Noonan syndrome, a human genetic disease, of which the pathogenic mechanism has not been precisely known yet. TE assessment holds significant promise as a model-free analysis method of reaction networks in molecular pharmacology and pathology.
-
- Computational and Systems Biology
- Genetics and Genomics
Root causal gene expression levels – or root causal genes for short – correspond to the initial changes to gene expression that generate patient symptoms as a downstream effect. Identifying root causal genes is critical towards developing treatments that modify disease near its onset, but no existing algorithms attempt to identify root causal genes from data. RNA-sequencing (RNA-seq) data introduces challenges such as measurement error, high dimensionality and non-linearity that compromise accurate estimation of root causal effects even with state-of-the-art approaches. We therefore instead leverage Perturb-seq, or high-throughput perturbations with single-cell RNA-seq readout, to learn the causal order between the genes. We then transfer the causal order to bulk RNA-seq and identify root causal genes specific to a given patient for the first time using a novel statistic. Experiments demonstrate large improvements in performance. Applications to macular degeneration and multiple sclerosis also reveal root causal genes that lie on known pathogenic pathways, delineate patient subgroups and implicate a newly defined omnigenic root causal model.