lncRNA read-through regulates the BX-C insulator Fub-1
Abstract
Though long non-coding RNAs (lncRNAs) represent a substantial fraction of the Pol II transcripts in multicellular animals, only a few have known functions. Here we report that the blocking activity of the Bithorax complex (BX-C) Fub-1 boundary is segmentally regulated by its own lncRNA. The Fub-1 boundary is located between the Ultrabithorax (Ubx) gene and the bxd/pbx regulatory domain, which is responsible for regulating Ubx expression in parasegment PS6/segment A1. Fub-1 consists of two hypersensitive sites, HS1 and HS2. HS1 is an insulator while HS2 functions primarily as an lncRNA promoter. To activate Ubx expression in PS6/A1, enhancers in the bxd/pbx domain must be able to bypass Fub-1 blocking activity. We show that the expression of the Fub-1 lncRNAs in PS6/A1 from the HS2 promoter inactivates Fub-1 insulating activity. Inactivation is due to read-through as the HS2 promoter must be directed toward HS1 to disrupt blocking.
Editor's evaluation
This study provides compelling evidence for the mechanism of an insulator element, which establishes boundaries between gene neighborhoods to allow proper gene regulation. In the fruit fly Drosophila melanogaster, the bithorax complex contains a series of Hox genes that determines segment identity. The authors show that transcriptional read-through of an bithorax insulator controls its activity and is used for proper patterning of the embryo.
https://doi.org/10.7554/eLife.84711.sa0Introduction
The vast majority of Pol II transcripts encoded by the genomes of multicellular animals correspond to long non-coding RNAs (lncRNAs), not mRNAs. Their wide distribution in the genome and the fact that their expression is often coordinated with genes in the immediate vicinity have led to the idea that they have functions in gene regulation and chromosome organization (Herman et al., 2022; Li and Fu, 2019; Núñez-Martínez and Recillas-Targa, 2022; Statello et al., 2021). Unlike the sequences of protein coding mRNAs, which are typically conserved across species, the primary sequences of lncRNAs are usually not very well conserved. However, some lncRNAs contain short sequence blocks that exhibit a relatively high degree of conservation, even though most of the lncRNA are divergent. In these instances, the lncRNA itself has regulatory activities. A classic example is the mammalian X chromosome inactivation lncRNA Xist. Conserved RNA sequence blocks within the Xist transcript function in recruiting Polycomb (PcG) silencing factors and targeting the transcript to the X chromosome (Jacobson et al., 2022; Lu et al., 2020; Pandya-Jones et al., 2020). Other lncRNAs, like the HoxA complex lncRNA HOTT1P, encode RNA sequences that recruit chromatin modifiers that help promote gene expression (Wang et al., 2011). In other cases, the lncRNA itself does not appear to have important functions. Instead, it is the regulatory elements (enhancers, silencers, and promoters) that control the expression of the lncRNA that are functionally important (Ibragimov et al., 2020). For example, the expression of the Myc gene is downregulated by a mechanism in which the promoter for the PVT1 lncRNA competes with Myc for physical interactions with a set of shared enhancers. In other cases, the transcription of the lncRNA appears to be the important regulatory function. Isoda et al. found that transcription of ThymoD lncRNA helps regulate the expression of Bcl11b, a gene that plays an important role in specifying T cell fate (Isoda et al., 2017). Transcription of the lncRNA induces the demethylation of recognition sites for the chromosomal architectural protein CTCF, enabling CTCF to bind to these sites and induce looping between the Bcl11b enhancers and the Bcl11b gene.
In the studies reported here, we have investigated the role of lncRNAs in regulating the chromatin organization and expression of the homeotic gene, Ultrabithorax (Ubx), in the Drosophila bithorax complex (BX-C). Ubx, together with abdominal-A (abd-a) and Abdominal-B (Abd-B), are responsible for specifying the identity of the nine parasegments (PS)/segments that form the posterior two-thirds of the fly (Maeda and Karch, 2015: Figure 1). Parasegment identity depends upon a series of nine regulatory domains that direct the temporal and tissue-specific patterns of expression of the three BX-C homeotic genes. Ubx is responsible for specifying parasegments PS5 (adult cuticle segment T3) and PS6 (adult cuticle segment A1). Its expression in these two parasegments is controlled by the abx/bx and bxd/pbx regulatory domains, respectively. abd-A expression in PS7-PS9 is directed by the iab-2–iab-4 regulatory domains, while Abd-B expression in PS10-14 is regulated by the iab-5–iab-9 regulatory domains. Each regulatory domain has an initiation element, a set of tissue-specific enhancers, and Polycomb Response Elements (PREs) (Iampietro et al., 2010; Maeda and Karch, 2015; Maeda and Karch, 2006). Early in development, a combination of maternal, gap, and pair-rule gene proteins interact with the initiation elements in each regulatory domain, and set the domain in either the ‘off’ or ‘on’ state. The BX-C regulatory domains are sequentially activated along the anterior–posterior axis of the embryo. For example, in PS5(T3) only the abx/bx domain is activated (all of the remaining BX-C domains including bxd/pbx are ‘off’) and it is responsible for regulating Ubx expression in this parasegment. Both abx/bx and bxd/pbx are turned on in PS6(A1); however, Ubx expression and parasegment identity depend on the bxd/pbx domain. Once the activity state of the domain is set in early embryos, it is remembered during the remainder of development by the action of Polycomb Group proteins (PcG: off) and Trithorax group proteins (Trx: on). Each domain also contains tissue- and stage-specific enhancers that drive the expression of its target homeotic gene in a pattern appropriate for proper development of the parasegment it specifies.
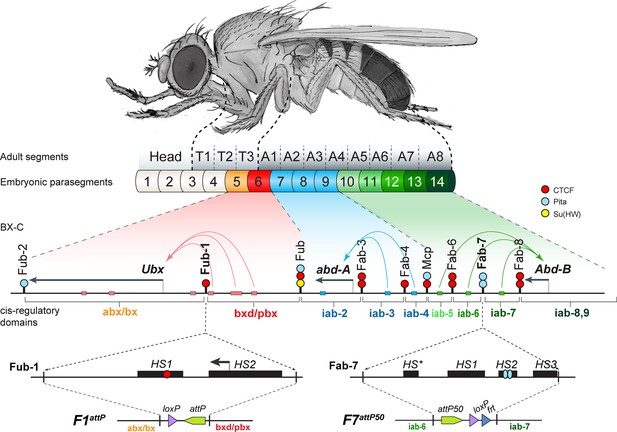
The organization of the genes and regulatory domains in BX-C.
The Drosophila melanogaster Bithorax complex (BX-C), which contains the Hox genes Ubx, abd-A, and Abd-B, is shown in relation to where these three genes are expressed in an embryo. There are nine cis-regulatory domains that are responsible for the regulation of Ubx (abx/bx and bxd/pbx domains), abd-A (iab-2–4 domains), and Abd-B (iab-5–7 and iab-8,9 domains), and for the development of parasegments 5–13 (PS)/segments (T3–A8). The anterior limit of expression of the three Hox gene is indicated by color coding: red: Ubx; blue: abd-A; green: Abd-B (reviewed in Maeda and Karch, 2015). The lines with colored circles mark chromatin boundaries. dCTCF, Pita, and Su(Hw) binding sites at the boundaries are shown as red, blue, and yellow circles/ ovals, respectively. Embryonic enhancers are indicated by pink, blue, and green bars on coordinate line. On the bottom of the figure, the molecular maps of the Fub-1 and Fab-7 boundaries are shown, including their deletions. Transposase/nuclease hypersensitive sites are shown as black boxes above the coordinate bar. The proximal and distal deficiency endpoints of the Fub-1 and Fab-7 deletions used in the replacement experiments are indicated by vertical lines. The attP, lox, and frt sites used in genome manipulations are shown as green, violet, and blue triangles, respectively.
In order to properly specify parasegment identity, the nine BX-C regulatory domains must be functionally autonomous. Autonomy is conferred by boundary elements (insulators) that flank each domain (Maeda and Karch, 2015). The role of boundaries in BX-C regulation is best understood in the Abd-B region of the complex. Mutations that inactivate Abd-B boundaries cause a gain-of-function (GOF) transformation in segment identity. For example, the Fab-7 boundary separates the iab-6 and iab-7 regulatory domains that are responsible for specifying PS11(A6) and PS12 (A7) identity, respectively (Gyurkovics et al., 1990). When Fab-7 is deleted, the iab-6 initiator activates the iab-7 domain in PS11(A6) and iab-7 instead of iab-6 drives Abd-B expression in this parasegment as well as in PS12(A7). This results in the duplication of the PS12/A7 segment. However, blocking crosstalk between adjacent regulatory domains is not the only function of BX-C boundaries. The three homeotic genes and their associated regulatory domains are arranged so that all but three of the domains are separated from their targets by one or more intervening boundaries. For this reason, all but two (Fub and Mcp) of the internal BX-C boundaries must also have bypass activity (Hogga et al., 2001; Kyrchanova et al., 2019a; Kyrchanova et al., 2023; Kyrchanova et al., 2019b; Kyrchanova et al., 2015). For example, Fab-7 has bypass activity and it mediates regulatory interactions between iab-6 and Abd-B. However, when Fab-7 is replaced by generic fly boundaries (e.g., scs or su(Hw)) or by BX-C boundaries that lack bypass activity (Mcp), these boundaries block crosstalk but do not support bypass (Hogga et al., 2001; Kyrchanova et al., 2017). As a consequence, Abd-B expression in PS11/A6 is driven by iab-5 not iab-6, and this results in a loss-of-function (LOF) phenotype.
The Ubx domain is delimited and subdivided by three boundaries called Fub-2, Fub-1, and Fub. Fub-2 and Fub-1 flank abx/bx domain, whereas bxd/pbx is flanked by Fub-1 and Fub (Figure 1; Bowman et al., 2014; Mateo et al., 2019). As Fub-1 separates enhancers in bxd/pbx regulatory domain from Ubx gene (Figure 1), it would have to be bypassed so that bxd/pbx can direct Ubx expression in PS6/A1. Unlike the Abd-B region of the complex where multiple boundaries are located between the iab-5 and the Abd-B promoter regulatory domain, there is only one boundary between bxd/pbx and the Ubx gene. As a consequence, the bypass mechanism might differ from that deployed elsewhere in BX-C. Here we show that segmentally regulated transcription of an lncRNA disrupts the blocking activity of Fub-1, enabling enhancers in the bxd/pbx regulatory domain to activate Ubx in PS6/A1 cells.
Results
The bxd/pbx regulatory domain: Boundaries and lncRNAs
The bxd/pbx regulatory domain controls Ubx expression in A1/PS6. This domain is off in more anterior parasegments (segments), while it is turned on in A1/PS6 and more posterior parasegments. The bxd/pbx domain is bracketed by the Fub boundary on the centromere distal side and Fub-1 on the centromere proximal side. The chromosomal architectural proteins CTCF, Pita, and Su(Hw) are associated with Fub in ChIP experiments, and like boundaries the Abd-B region of BX-C Fub deletions result in a GOF transformation of PS6(A1) into PS7(A2). The Fub-1 boundary has only been defined molecularly. ATAC-seq mapping in nuclear cycle 14 embryos indicates that this boundary includes two chromatin-specific transposase hypersensitive regions: HS1 ~200 bp and HS2 ~300 bp (Figure 1; Hannon et al., 2017). In ChIP experiments, Fub-1 is bound by the chromosomal architectural proteins CTCF, CP190, Zw5, Pita, and Elba and marks the border of a Polycomb histone H3K27me3 domain in PS5/T3 cells (Bowman et al., 2014, p. 27; Fuda et al., 2015; Harrison et al., 2011; Ueberschär et al., 2019; Zolotarev et al., 2016; Figure 1—figure supplement 1). In addition, RNA Pol II and the histone mark for active transcription, H3K4me3, both map to Fub-1. Consistent with the results described below, the signal for H3K4me3 in PS7 cell ChIPs is greater than that observed in mixed ChIPs (Bowman et al., 2014, p. 27). In between Fub-1 and the Ubx promoter, there are two prominent Zelda peaks and a putative enhancer (Figure 1—figure supplement 1; Harrison et al., 2011).
Besides directing the expression of Ubx in PS6(A1) and more posterior parasegements, the bxd/pbx domain also controls the expression of two lncRNAs, bxd and Fub-1 HS2, Fub-1HS2. Pease et al. found that the bxd lncRNA initiates from a promoter close to the pbx enhancer and extends into the region located in between Fub-1 and the Ubx promoter (Pease et al., 2013). The bxd lncRNA is first detected at the blastoderm stage as a broad band that extends from PS6/A1 to PS12/A7. Between the onset of gastrulation and stage 10 bxd lncRNA expression resolves into a series of eight stripes of differing intensity that span PS6/A1–PS12/A7. Pease et al. also detected a second bxd/pbx-dependent lncRNA, Fub-1HS2. Like bxd lncRNA, it is expressed in PS6 (A1) and more posterior parasegments. This lncRNA is encoded by sequences located between Ubx and Fub-1, including sequences in Fub-1 HS1, and based on the in situ experiments of Pease et al., it likely originates from Fub-1 HS2. Fub-1HS2 shares exon sequences with the bxd lncRNA. However, the Fub-1HS2 lncRNA is detected in PS6/A1 and more posterior segments after the bxd lncRNA disappears.
As has been reported for the regulatory elements associated with other lncRNAs, the Fub-1 hypersensitive sites are evolutionarily conserved. As shown in Figure 1—figure supplement 2, key sequence blocks within HS1 and HS2 are detected not only in other members of the Sophophora subgenus, but also in Drosophila virilis, a distantly related member of the Drosophila subgenus. The conserved blocks in HS1 include the dCTCF recognition site, while in HS2 the conserved blocks include several GAGA motifs that correspond to binding sites for the GAGA factor (GAF) as well as the predicted transcription start sites for the Fub-1HS2 lncRNA.
Fub-1 subdivides the Ubx regulatory domains
These observations, together with the studies of Bowman et al., showed that Fub-1 marks the border of a Polycomb histone H3K27me3 domain in PS5 cells would predict that Fub-1 delimits the endpoint of two adjacent TADs. The centromere distal TAD would encompass the bxd/pbx regulatory domain and end at Fub boundary that is located downstream of the abd-A transcription unit (Bender and Lucas, 2013; Bowman et al., 2014). The centromere proximal TAD would encompass the entire Ubx transcription unit and end at a predicted Pita/CP190 boundary, Fub-2, that is located between the 3′ end of the Ubx gene and the 5′ end of modSP.
To test these predictions, we used Micro-C to examine the TAD organization of Ubx and its two regulatory domains in 12–18 hr embryos. As shown in Figure 2, both regulatory domains (plus the Ubx transcription unit) are encompassed in a large TAD that extends from Fub-2 on the centromere proximal side of Ubx to Fub on the distal side. Within this large TAD, there is an ~50 kb sub-TAD that spans bxd/pbx and is delimited by Fub-1 on the left and Fub on the right (Figure 2B and C, black arrow). This bxd/pbx sub-TAD includes several smaller chromosomal segments that exhibit a high density of internal contacts (HDICs). The endpoint for one of these HDIC domains maps to Fub-1, while the other endpoint maps to the bxd PRE. There is a second HDIC domain linking Fub-1 to sequences just upstream of the Ubx promoter. The Ubx promoter is also linked to the bxd PRE by a lower density of internal contacts domain (LDIC) that is marked at the apex by an interaction dot (*). The bxd PRE also forms an interaction dot (>) with the bx PRE as does the Ubx promoter (x). Interestingly PREs in other developmental loci have recently been shown to interact with each other and also function as ‘tethering’ elements helping to link distant enhancers to their target genes (Batut et al., 2022; Eagen et al., 2017; Ibragimov et al., 2022; Levo et al., 2022).
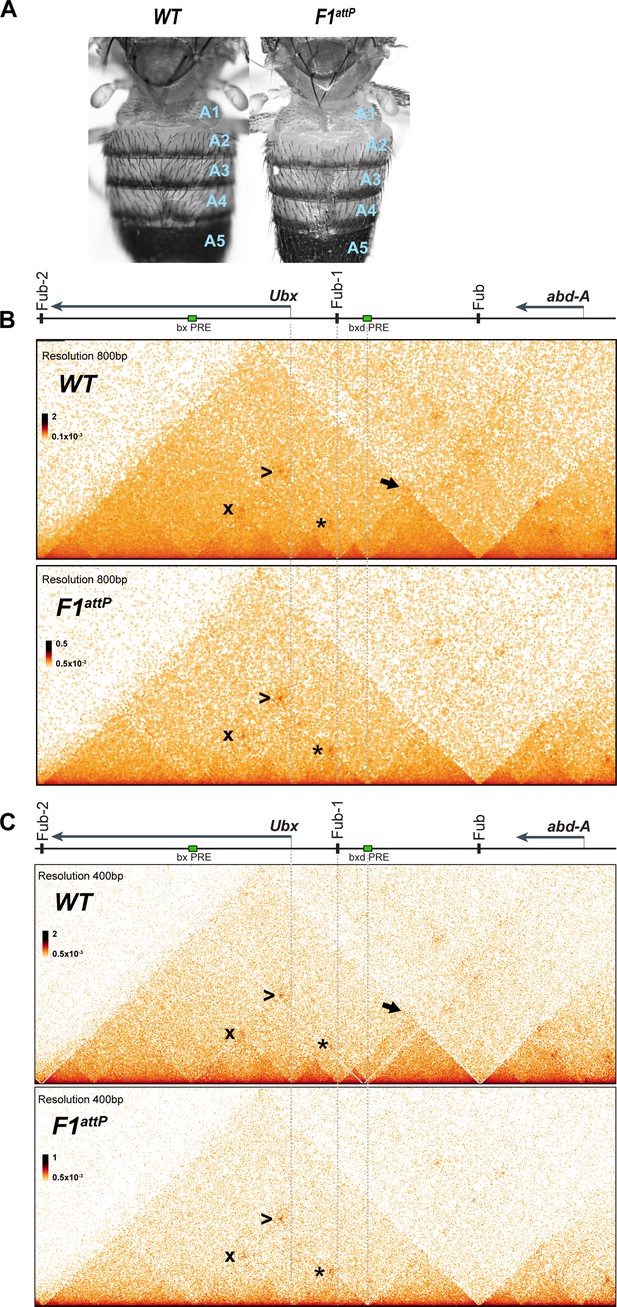
Chromatin topology in bxd/pbx domain in WT and in Fub-1 deletion.
(A) Morphology of the abdominal segments of WT and F1attP flies. (B) Micro-C contact map of the WT and F1attP 12–18 hr embryos at 800 bp resolution. The black arrow points to a sub-TAD formed by Fub-1 and Fub boundaries. The Ubx promoter is linked to the bxd PRE by a lower density of internal contacts domain (LDIC) that is marked at the apex by an interaction dot (*). The bxd PRE forms an interaction dot (>) with the bx PRE as does the Ubx promoter (x). (C) Micro-C contact map of the WT and F1attP 12–18 hr embryos at 400 bp resolution.
Fub-1 is not required to block crosstalk between abx/bx and bxd/pbx
A BX-C boundary in the location of Fub-1 would be expected to have two regulatory functions. One would be blocking crosstalk between abx/bx and bxd/pbx while the other would be bypass so that enhancers in the bxd/pbx domain would be able to drive Ubx expression in PS6/A1. To test for blocking activity, we generated a 1168 bp deletion (F1attP) that retains an attP site for boundary replacement experiments (Figure 1, Figure 1—figure supplement 3). Deletions of boundaries elsewhere in BX-C typically result in GOF transformations. This transformation arises because initiators in the domain centromere proximal (Figure 1, right) to the boundary are able to active the domain distal to the boundary (Figure 1, left) in a parasegment in which the distal domain would normally be silent (Barges et al., 2000; Bender and Lucas, 2013; Gyurkovics et al., 1990; Karch et al., 1994; Postika et al., 2021). For this reason, we anticipated that the bxd/pbx domain would be inappropriately activated by initiators in abx/bx in PS5, and in adults we would observe a GOF transformation of T3 (PS5) toward A1 (PS6). Unexpectedly, however, F1attP flies do not show any evidence of a GOF transformation and their morphology is indistinguishable from wild type (WT) (Figure 2A).
A plausible explanation for this result is that there is a nearby element that can substitute for Fub-1 and block crosstalk between abx/bx and bxd/pbx. Figure 2B and C show that the sub-TAD linking Fub-1 to Fub that encompassed the bxd/pbx regulatory domain disappears in the Fub-1 deletion. However, a new sub-TAD that includes Fub does not appear to be formed. Instead, the element located just upstream of the Ubx promoter forms a sub-TAD domain with the bxd PRE (see interaction dot in Figure 2B and C [*] at the apex). It may be that this topological configuration is sufficient to block crosstalk between initiators in abx/bx and bxd/pbx when the activity state of these domains in T3 (PS5) is set during early embryogenesis.
The Fub-1 element does not block enhancers in bxd/pbx from activating reporters
Although Fub-1 defines one endpoint of a TAD and marks the border for the PcG H3K27me3 histone mark in PS5 nuclei, the finding that Fub-1 deletions have no phenotype raises the possibility that it is actually ‘within’ the bxd/pbx regulatory domain. If this is the case, then reporters inserted between Fub-1 and the element just upstream of the Ubx promoter should respond to bxd/pbx enhancers, but be insulated from enhancers in the abx/bx regulatory domain. To test this possibility, we analyzed the pattern of expression of reporters inserted into the F1attP site with and without the Fub-1 boundary.
We first examined the DsRed reporter used to mark the Fub-1 deletion in DsRed-F1attP. Figure 3 shows that DsRed is expressed throughout the posterior ~2/3rds of Fub-1 deletion embryos, with an anterior limit corresponding to the anterior border of PS6/A1. Thus, as expected from the lack of phenotypic effects of the Fub-1 deletion, the reporter is insulated from the abx/bx domain, but is subject to regulation by the bxd/pbx domain. We next introduced the 1168 bp Fub-1 fragment together with a GFP reporter flanked by frt sites back into the DsRed-F1attP platform (Figure 1—figure supplement 3). In the resulting insert, the Fub-1 element is flanked by DsRed on the proximal Ubx side and GFP on the distal bxd/pbx side. The reporters were then excised individually to give DsRed-Fub-1 and Fub-1-GFP (Figure 1—figure supplement 3). As shown in Figure 3, the anterior border of expression of both DsRed and GFP corresponds to the PS6/A1 as is observed for DsRed when the Fub-1 element is deleted. Thus Fub-1 is unable to block the bxd/pbx enhancers from activating the DsRed reporter in PS6/A1 cells (and more posterior parasegments/segments), and this reporter is also ‘located’ within the bxd/pbx regulatory domain.
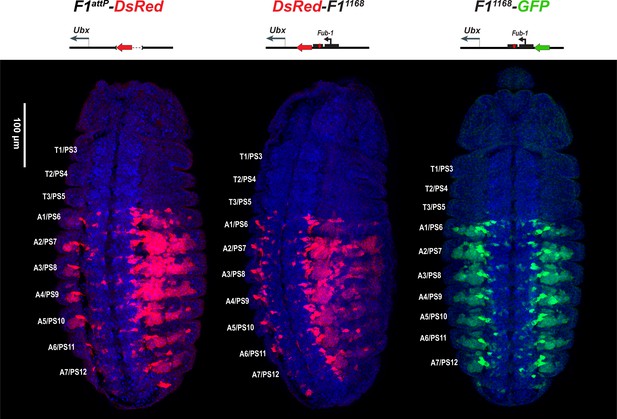
DsRed and GFP markers trap enhancer activity in Fub-1 replacements.
DsRed (in red) and GFP (in green) expression in stage 14 embryos in the Fub-1 deletion and in two replacements as indicated. DAPI was used to stain nuclei (in blue). DsRed expression in F1attP-DsRed begins in PS6/A1. In DsRed-F11168 and F11168-GFP, expression patterns of both markers are limited to PS6/A1-PS13/A8, consistent with the idea that the Ubx promoter region can function to demarcate the abx/bx and bxd/pbx domains.
These findings fit with previous enhancer trap studies that showed that P-element insertions around the Ubx promoter displayed two distinct patterns of expression. An enhancer trap located 13 bp upstream of the Ubx transcription start site had a PS5/T3 anterior border, indicating that it is regulated by the abx/bx domain. In contrast, an enhancer trap located 196 bp upstream of the start site had an anterior limit of expression shifted to PS6/A1 (McCall et al., 1994). Taken together with the experiments described in the previous section, it would appear that the Ubx promoter region is sufficient for the functional autonomy of the abx/bx and bxd/pbx regulatory domains under laboratory growth conditions.
Full-length Fub-1 replacements of Fab-7 do not block crosstalk between iab-6 and iab-7
The results described above are unexpected for several reasons. First, Fub-1 defines the proximal endpoint of a TAD that extends to Fub and encompasses the bxd/pbx regulatory domain. Second, it also corresponds to the proximal border of the PcG H3K27me3 histone mark in PS5 nuclei where abx/bx should be ‘on’ and bxd/pbx should be ‘off.’ Third, both of the Fub-1 sub-elements, HS1 and HS2, are evolutionarily conserved. In spite of these properties, deletion of Fub-1 does not result in a detectable GOF transformation of T3/PS5 into A1/PS6. This is likely explained by the presence of the boundary element upstream of the Ubx promoter, which is able to block crosstalk between initiators in abx/bx and pbx/bxd. However, this would not explain why Fub-1 fails to block the pbx/bxd enhancers from activating a DsRed reporter when interposed between the enhancers and the reporter. These observations raise a novel possibility: even though Fub-1 has many of the characteristic chromosome architectural functions of boundary elements, it differs from other boundaries in flies and other organisms in that it lacks insulating activity.
To test whether Fub-1 lacks insulating activity, we took advantage of the Fab-7attP50 (F7attP50) replacement platform (Figure 1; Wolle et al., 2015). In this platform, the Fab-7 boundary was deleted, and an attP site introduced in its place. The deletion of Fab-7 fuses the iab-6 and iab-7 regulatory domains, enabling parasegment-specific initiation elements in iab-6 to ectopically activate iab-7 (Karch et al., 1994; Wolle et al., 2015). As a consequence, iab-7 drives Abd-B expression in PS11, transforming PS11/A6 into a duplicate copy of PS12/A7 (Figure 4B and C). The attP site in the F7attP50 can be used to insert any sequences of interest to test for two different boundary functions. The first is blocking crosstalk between iab-6 and iab-7, while the second is supporting bypass so that iab-6 can regulate Abd-B in PS11/A6.
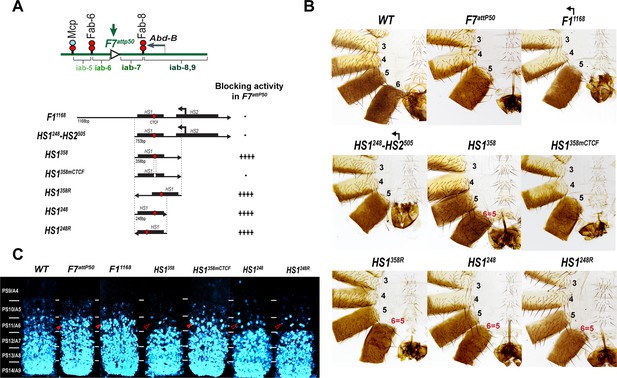
Testing boundary activity of Fub-1 sequences.
(A) Top: schematic presentation of Fab-7 substitution. Bottom: Fub-1 fragments used in the replacement experiments. On the right side, the insulator activity with the various fragments in the F7attP50 insertion site in adults as judged from cuticle preps. The number of ‘+’ signs reflects the strength of insulator activity, where ‘++++’ is full blocking, and ‘-’ is lack of detectable blocking activity, respectively. Designations are the same as described in Figure 1. (B) Morphology of the male abdominal segments (numbered) in F11168, HS1248-HS2505, HS1358, HS1358mCTCF, HS1358R, HS1248, and HS1248R replacements. (C) Abd-B expression in Fab-7 replacement embryos. Each panel shows a confocal image of the embryonic CNS of stage 15 embryos stained with antibodies against Abd-B (cyan). The filled red arrowheads show morphological features indicative of gain-of-function (GOF) transformations. The empty red arrowheads show the signs of the loss-of-function (LOF) transformation, which is directly correlated with the boundary function of tested DNA fragments. The WT expression pattern of Abd-B in the embryonic CNS is characterized by a stepwise gradient of increasing protein level from PS10/A5 to PS14/A8. In F7attP50 embryos, Abd-B expression level in PS11/A6 is roughly equal to that in PS12/A7, indicating that iab-7 drives Abd-B expression in PS11/A6 (GOF phenotype). Consistent with the adult phenotype, in F11168 and HS1248-HS2505 Abd-B expression in PS11/A6 is the same as in F7attP50. The Abd-B expression pattern in HS1358 and HS1248 replacement is also consistent with the adult cuticular phenotypes: Abd-B expression is reduced in both PS10/A5 and PS11/A6 (LOF phenotype) compared with WT. In contrast, mutation of dCTCF site in HS1358mCTCF results in the loss of blocking activity and Abd-B expression pattern similar to F7attP50.
In the first experiment, we used the F7attP50 replacement platform to test the blocking activity of a 1168 bp sequence spanning the entire deletion, Fub-11168, and a 753 bp sequence including only hypersensitive sites, HS1248-HS2505 (R6.22: 3R:16748578–16749330) inserted in the same 5′→3′ orientation as the endogenous Fub-1 element. Figure 4B and Figure 4—figure supplement 1 show that males carrying either the 1168 bp or the 753 bp replacements lack A6 just like the starting F7attP50 deletion. This result indicates that these two Fub-1 fragments do not prevent crosstalk between iab-6 and iab-7. The HS1248-HS2505 replacement GOF phenotype is reflected in the pattern of Abd-B expression in the embryonic CNS that closely matches that of the starting F7attP50 deletion (Figure 4C). We also inserted the two Fub-1 fragments in the reverse orientation; however, in this case also neither of the fragments rescued the GOF phenotype of the F7attP50 deletion (Figure 5).
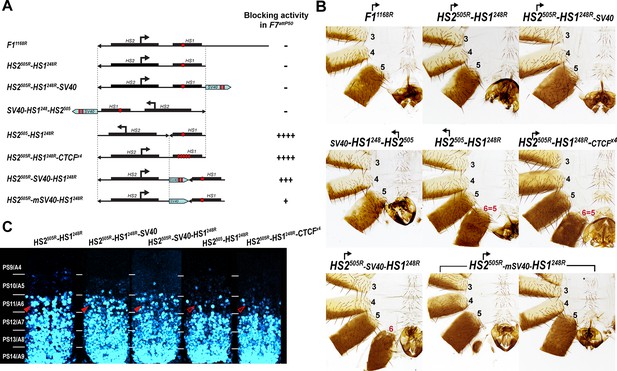
Read-through transcription inactivates HS1 insulator.
(A) Schematic representation of sequences tested in F7attP50. SV40 terminator is shown as a light blue arrow, and poly(A) sites are marked as vertical red lines. All other designations are the same as described in Figure 4. (B) Morphology of the male abdominal segments (numbered) of Fab-7 replacements. (C) Abd-B expression in CNS of Fab-7 replacement embryos. Embryos were stained and marked as in Figure 4.
HS1 has boundary activity
Previous replacement experiments have shown that dCTCF-associated BX-C boundaries like Mcp are able to block crosstalk between iab-6 and iab-7 (Postika et al., 2018). For this reason, we wondered whether HS1 alone has blocking activity. We used two different HS1 fragments to test this possibility. One was 358 bp (R6.22: 3R: 16748578–16748935) and included all of the HS1 sequences plus the sequences located between HS1 and HS2. The other, a 248 bp fragment (R6.22: 3R: 16748578–16748825), contains only the HS1 region. These fragments were inserted in both the forward and reverse orientations. We also tested a 505 bp fragment spanning the HS2 (R6.22: 3R: 16748826–16749330) in both the forward and reverse orientations (Figure 4—figure supplement 2). While HS2 lacks blocking activity (Figure 4—figure supplement 1 and Figure 4—figure supplement 2), Figure 4B shows that both of the HS1 fragments block crosstalk between iab-6 and iab-7 and rescue the GOF transformation of A6 into A7. The rescuing activity is orientation independent. In all four cases, however, the morphology of the A6 segment resembles that normally observed in A5, not A6. Instead of the banana-shaped A6 sternite observed in WT, the A6 sternite in HS1 replacements has a quadrilateral shape and is covered in bristles just like the A5 sternite (Figure 4). A similar LOF A6 toward A5 transformation is evident in A6 tergite, which is covered in trichome hairs like A5 tergite (Figure 4—figure supplement 1). This LOF phenotype is observed when the Fab-7 replacements have insulating activity but do not support bypass (Hogga et al., 2001; Kyrchanova et al., 2017). Note, however, that HS1 replacements cannot block iab-5 domain from activating Abd-B, and A5 segment identity is not changed. This is likely due to the fact that Fab-6 boundary can ‘bypass’ this intervening insulator and target iab-5 enhancers to Abd-B promoter (for detailed review, see Kyrchanova et al., 2015). Consistent with the adult phenotypes, Abd-B expression in the embryonic CNS is suppressed in A6 by the HS1 fragments (Figure 4C).
dCTCF recognition sequences were found to be required for the blocking activity of other CTCF-associated BX-C boundaries (Kyrchanova et al., 2017; Kyrchanova et al., 2016, p. 8). This is also true for the dCTCF site in HS1. Figure 4 shows that a mutation in the HS1 dCTCF binding site completely disrupts insulating activity. Like the starting F7attP50 platform, the adult HS1358mCTCF males lack the A6 segment, indicating that PS11/A6 is fully transformed into a copy of PS12/A7 (Figure 4—figure supplement 1). Consistent with a loss of blocking activity, the pattern of Abd-B expression in HS1358mCTCF embryos is similar to that of F7attP50 (Figure 4C).
Read-through transcription from HS2 abrogates HS1 insulator function
The finding that fragments containing only HS1 have insulating activity, while the full-length Fub-1 fragment does not, suggests that when HS2 is present it inactivates the HS1 boundary. One likely mechanism is transcriptional read-through. Studies on the induction of the chicken lysozyme gene by bacterial lipopolysaccharides (LPS) by Lefevre et al. showed that LPS treatment activated the transcription of an lncRNA through a CTCF boundary (Lefevre et al., 2008). Read-through transcription resulted in the inactivation of the boundary enabling distal enhancers to bypass the boundary and turn on the lysozyme gene.
To test this hypothesis, we inserted a 229 bp SV40 transcription terminator in between HS2 and HS1 to give the HS2505R-SV40-HS1248R replacement (Figure 5A, Supplementary file 1a). Unlike the starting HS2505R-HS1248R line, an A6-like segment is present in HS2505R-SV40-HS1248R males (Figure 5B and Figure 4—figure supplement 1). However, blocking is not fully restored to the level of HS1248R alone. In HS2505R-SV40-HS1248R males, the A6 tergite is slightly reduced in size while the sternite is absent. In the embryonic CNS, Abd-B expression in PS11 is clearly elevated, but less so than that observed in either HS2505R-HS1248R or F7attP50 (Figure 5C and Figure 5—figure supplement 1). To confirm that the partial reactivation of blocking activity is due to a reduction in transcriptional read-through, we generated a similar construct, HS2505R-mSV40-HS1248R (Supplementary file 1a), in which the polyadenylation sequences in the SV40 fragment were mutated. In contrast to HS2505R-SV40-HS1248R, the insulating activity of HS2505R-mSV40-HS1248R is substantially compromised and only a residual A6 tergite is observed (Figure 5B and Figure 4—figure supplement 1).
In these experiments, the SV40 termination element will not only suppress transcription through HS1, but also into the neighboring iab-7 regulatory domain. To rule out the possibility that the SV40 element ‘rescues’ the blocking activity of HS1 by reducing transcription into the iab-7 regulatory domain, we placed it downstream of HS1 instead between HS2 and HS1. Figures 5B and S4 and 4 show that just like HS2505R-HS1248R, HS2505R-HS1248R-SV40 has no boundary function: A6 is absent in males carrying the replacement, indicating that the cells in PS11/A6 have assumed a PS12/A7 identity. The Abd-B expression pattern in the embryonic CNS also closely matches that of HS2505R-HS1248R line (Figure 5C). Similar results were obtained when the same construct (SV40-HS1249-HS2505) was inserted in the forward orientation so that the HS2 element is ‘pointing’ toward the iab-6 domain (Figure 5 and Figure 4—figure supplement 1).
To provide further evidence that transcriptional read-through from HS2 is responsible for disrupting the blocking activity of HS1, we generated a replacement, HS2505-HS1248R, in which the 5′→3′ orientation of HS2 was inverted with respect to HS1. If transcription from HS2 is unidirectional, then the blocking activity of HS1 should be unaffected when HS2 is ‘pointing’ away from HS1. Consistent with this prediction, HS1 retains blocking activity in HS2505-HS1248R. Figure 5C shows that the pattern of Abd-B expression in the embryonic CNS in both PS10 and PS11 is reduced by HS2505-HS1248R, while the cuticle phenotype in adult males resembles HS1248 (Figure 5B). Taken together, these results show that read-through transcription from HS2 is responsible for the inactivation of HS1 boundary function.
Transcription from HS2 promoter cannot overcome five dCTCF sites
Transcriptional read-through by RNA Polymerase II (Pol II) could disrupt HS1 boundary activity by temporarily displacing dCTCF as well as other boundary associated factors. If this is the case, it seemed possible that the presence of additional dCTCF sites in HS1 would counteract the effects of transcription from HS2 either by helping to maintain boundary function as Pol II read-throughs or by inhibiting Pol II elongation. To test this possibility, we inserted four additional dCTCF sites in an HS1 Sph I restriction site located close to the endogenous dCTCF site. Figure 5B shows that the addition of four dCTCF sites (HS2505R-HS1248R-CTCFx4) is sufficient to rescue the blocking activity of HS1. As observed for HS1 alone, HS2505R-HS1248R-CTCFx4 males have a tergite and sternite whose morphology resembles that seen in A5. Likewise, the pattern of Abd-B expression in the CNS in HS2505R-HS1248R-CTCFx4 is similar to that observed for HS248 (Figure 5C).
Segmental regulation of the HS1 boundary by HS2-dependent transcriptional read-through
Taken together, the findings in the previous sections suggest that Fub-1 likely functions as a boundary in its endogenous context; however, its boundary activity is segmentally regulated by transcriptional read-through from HS2. This model makes two predictions. First, the activity of the HS2 promoter should be controlled by the bxd/pbx regulatory domain. Second, when transcriptional read-through is prevented, Fub-1 blocking activity would be expected to interfere with Ubx regulation by the bxd/pbx regulatory domain. We have tested these predictions.
HS2 promoter activity is controlled by the bxd/pbx regulatory domain
In order to test the first prediction, we used smFISH probes spanning a 1983 bp region in the bxd domain located just proximal to Fub-1 (Figure 6A, Supplementary file 1b). As noted above, Pease et al. found that there are two distinct ‘sense’ lncRNAs, bxd and Fub-1HS2, expressed under the control of the bxd/pbx regulatory domain that could potentially read-through HS1 and be complementary to sequences in the region between HS1 and the Ubx promoter. For this reason, transcripts originating from the HS2 promoter and extending through HS1 toward the Ubx promoter cannot be unambiguously identified as being derived from HS2 in WT using probes proximal to (downstream of) Fub-1.
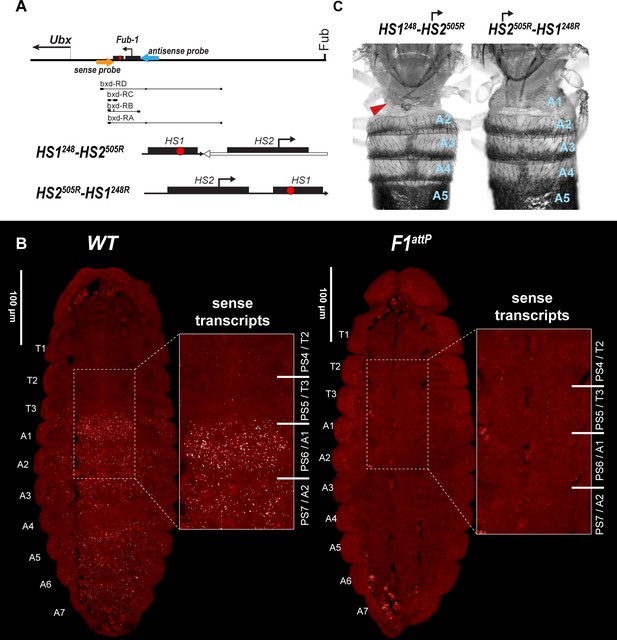
HS2 drives transcription in tissue-specific manner.
(A) Schematic presentation of bxd domain with HS1248-HS2505R and HS2505R-HS1248R replacements. The smFISH strand-specific probes are shown as orange and light blue arrows (Supplementary file 1b and c). All other designations are the same as described in Figure 1. Characterized bxd lncRNA transcripts are presented under coordinate line. (B) smFISH of stage 14 embryos of the indicated genotype with a probe that targets sense strand just proximal to Fub-1. In WT embryos Fub-1HS2 sense (distal to proximal) transcripts were detected from PS6/A1 through PS12/A7. By contrast, in Fub-1 deletion (F1attP) Fub-1HS2 transcripts were not detectable. (C) Morphology of the abdominal segments of HS1248-HS2505R and HS2505R-HS1248R flies. The red arrow shows the signs of the loss-of-function (LOF) phenotype: complete reduction of A1 segment and the appearance of postnatal tissue in its place.
As shown in Figure 6—figure supplement 1, we detected a broad band of sense transcripts in the posterior region of both WT and F1attP embryos as early as nuclear cycle 13. In WT, the smFISH probe is expected to hybridize to both Fub-1HS2 and bxd lncRNAs. In contrast, since the Fub-1HS2 promoter is deleted in F1attP embryos, only bxd lncRNAs should be detected. As would be expected if both bxd and Fub-1HS2 lncRNAs are expressed at this stage in WT embryos, the intensity of the smFISH signal is higher in WT compared to F1attP. During gastrulation, the broad band seen at nuclear cycle 13 resolves into a series of stripes of near equal width and intensity extending toward the posterior of the embryo (Figure 6—figure supplement 2). At this stage, the difference in the smFISH signal between WT and F1attP is even more striking than in pre-cellular blastoderm embryos. At around stage 11 of embryogenesis, the transcription of bxd lncRNAs seems to be shut off as we no longer detect lncRNAs complementary to the probe in F1attP embryos (Figure 6—figure supplement 3). In contrast, in WT, lncRNAs complementary to the probe are readily detected and are expressed in a pattern that resembles that of Ubx RNA, except that there is no staining in PS5/T3 (Figure 6—figure supplement 3). Thus, at this stage only the Fub-1HS2 promoter seems to be active. Like the DsRed and GFP reporters described above (Figure 3), Fub-1HS2 transcripts are detected in PS6/A1 through PS12/A7 (Figure 6—figure supplement 3). As shown in Figure 6B in stage 14 embryos, Fub-1HS2 transcripts are detected in the epidermis in PS6–PS12, though the highest level is in PS6. This pattern of expression indicates that the HS2 promoter is regulated by the bxd/pbx domain, while it is insulated from regulatory elements in the abx/bx domain.
Transcriptional read-through is required to abrogate HS1 blocking activity in PS6/A1
The second prediction is that transcriptional read-through from HS2 into HS1 is required to relieve the blocking activity of HS1, enabling regulatory interactions between the bxd/pbx domain and Ubx in PS6/A1.
To test this prediction, we generated two different Fub-1 replacements in which the 5′→3′ orientation of the HS2 element was reversed so that it would generate ‘antisense’ transcripts. In the first, we inserted a fragment, HS1248-HS2505R, into F1attP that contains both Fub-1 hypersensitive sites (Figure 6A). However, the 5′→3′ orientation of HS2 in this fragment was reversed so that it is pointing away from HS1. In this case, transcripts expressed from the HS2 promoter would extend into the bxd/pbx domain, instead of transcribing through HS1. In the second, we inserted a Fub-1 fragment in F1attP containing both hypersensitive sites, HS2505R-HS1248R, but in the reverse orientation so that transcription from HS2 would read-through HS1 toward the bxd/pbx regulatory domain (Figure 7A). As shown in Figure 6C, the morphology of the A1 segment in HS2505R-HS1248R replacement flies is similar to that in WT. In this replacement transcripts originating in HS2 read through HS1 into the bxd/pbx regulatory domain. A different result is obtained for the replacement, HS1248-HS2505R, in which the HS2 promoter is directed away from HS1. HS1248-HS2505R flies show evidence of an LOF transformation (Figure 6C). This replacement transforms the first abdominal segment into the third thoracic: the first abdominal tergite is reduced or absent; in addition, the posterior third thoracic segment is partly transformed to a posterior second thoracic segment: some flies have enlarged and/or pointing downward halters (Figure 6C and Figure 6—figure supplement 4). These phenotypes are known as bithoraxoid (bxd) and postbithorax (pbx), respectively (Lewis, 1963). HS1248-HS2505R homozygotes also display sterility. Homozygous flies showed very low viability, and we were able to generate homozygote larvae and flies only when growing heterozygotes on rich medium (Backhaus et al., 1984).
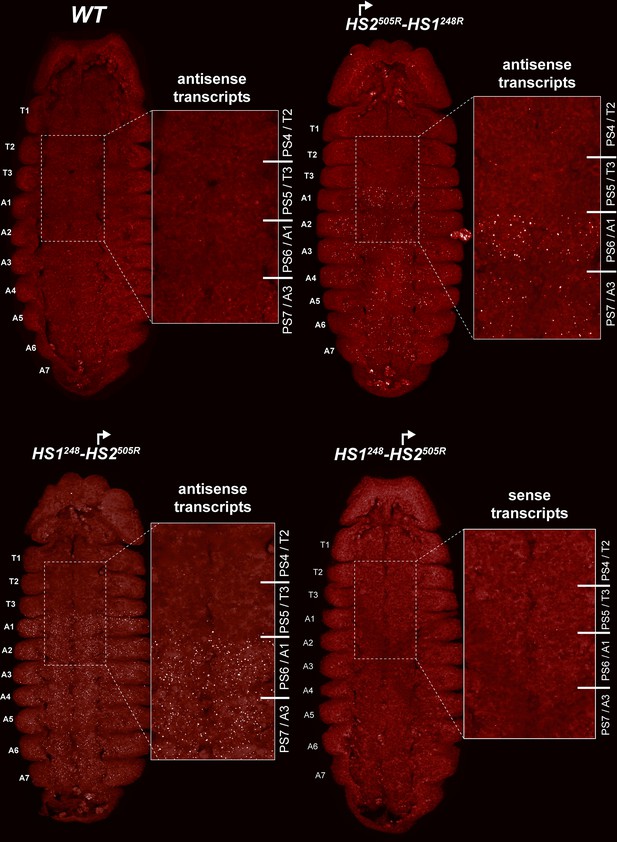
HS2 drives transcription in a tissue-specific manner.
smFISH of stage 14 embryos of indicated genotype with a probe that targets antisense strand just distal to Fub-1. In WT embryos, no antisense (proximal to distal) transcripts were detected. By contrast, in HS1248-HS2505R and HS2505R-HS1248R antisense transcripts are detected from PS6/A1 through PS12/A7. Note that the intensity of smFISH signal is higher in HS1248-HS2505R than in HS2505R-HS1248R. In HS1248-HS2505R embryos, Fub-1HS2 sense (distal to proximal) transcripts were not detected, indicating that HS2 drives transcription only in one direction.
HS1248-HS2505R mutants also have an LOF phenotype at the larval stage. As shown in Figure S11, wild-type larvae have 11 denticle belts marking the anterior half of each of the thoracic and abdominal segments; these are visible as black dots. In WT, T3 has 2–3 rows of small denticles, whereas in A1 there are 3 anterior rows of large and 2 rows of small denticles. In the HS1248-HS2505R replacement, the A1 band of denticles is narrower and irregular, indicative of an LOF transformation of A1 toward T3 (Figure 6—figure supplement 5). We also observe a reduction in the expression of Ubx in the posterior compartment of the haltere discs compared to WT (Figure 6—figure supplement 6).
Since the HS2 promoter is inserted in the reverse orientation in both HS1248-HS2505R and HS2505R-HS1248R, it is expected to generate antisense transcript in response to regulatory elements in bxd/pbx. To test this prediction, we generated antisense smFISH probes spanning a 1463 bp bxd sequence located just distal to Fub-1 (Figure 6A, Supplementary file 1c). Figure 7 shows that as expected antisense transcripts complementary to the smFISH probes are not detected in WT embryos. In contrast, segmentally restricted antisense transcripts are expressed in both of the Fub-1 replacements. Like Fub-1HS2 sense transcripts in WT (Figure 6), the antisense transcripts are detected in PS6/A1 through PS12/A7 in HS1248-HS2505R and HS2505R-HS1248R. The transcripts levels in HS1248-HS2505R and HS2505R-HS1248R embryos are not, however, equivalent. The signal is noticeably greater in HS1248-HS2505R than it is in HS2505R-HS1248R. This could reflect the fact that the smFISH probes are complementary to sequences immediately adjacent to HS2 in the HS1248-HS2505R replacement, whereas the polymerase must traverse HS1 in the HS2505R-HS1248R replacement. Alternatively, since HS1 is located between the HS2 promoter and the bxd/pbx regulatory domain, it may attenuate regulatory interactions, and at least the initial activation in PS6/A1 may depend upon the enhancers thought to be located between the Ubx promoter and Fub-1.
In order to test whether HS2 drives transcription in both directions, we assayed HS1248-HS2505R with the probe targeting sense strand. Figure 7 shows that, unlike WT (Figure 6), sense transcription is not detectable when HS2 is inverted. This result indicates that the lncRNA promoter is situated in HS2 and drives transcription only in one direction.
Discussion
While a very large percentage of the Pol II transcripts in multicellular eukaryotes are lncRNAs, what roles they play in the expression of coding mRNAs and in development is largely unknown. Here we have investigated the functional properties of an lncRNA gene, Fub-1HS2, that is located in the bxd/pbx regulatory domain of Drosophila BX-C. As has been observed for many other lncRNA genes, the RNA sequences encoded by Fub-1HS2 are not well conserved and it is the functional properties of the two Fub-1HS2 regulatory elements, HS1 and HS2, that are important. We show here that HS1 is a dCTCF-dependent boundary element, while HS2 is a developmentally regulated promoter that directs transcription through HS1 inactivating its boundary function in parasegments that require Ubx function.
The arrangement of the three homeotic genes and the nine parasegment-specific regulatory domains in the Drosophila BX-C complicates the coordination between the 3D organization of the complex and the requirements for regulatory interactions. In order to specify segment identity, the nine regulatory domains are segregated into topologically independent loops by boundary elements. This physical organization helps to block crosstalk between initiation elements in adjacent domains in the early embryo, while later in development it helps restrict the spread of PcG silencing from inactive to active domains (Kyrchanova et al., 2015; Maeda and Karch, 2015). However, in order to direct the proper expression of their target homeotic gene, all but three of the regulatory domains must be able to bypass one or more intervening boundary elements. In the case of the Ubx gene, the bxd/pbx regulatory domain is separated from its target promoter by the Fub-1 boundary and by a second boundary element located close to the Ubx promoter.
The Fub-1 boundary is subdivided into two elements, HS1 and HS2. HS1, like other boundaries in BX-C, has a dCTCF site, is bound by dCTCF in vivo, and is able to function as a generic insulator. While HS2 may contribute to the boundary function of Fub-1, it does not have insulating activity on its own. Instead, it has promoter activity and can function as an enhancer trap. In its normal context, the HS2 promoter is controlled by the bxd/pbx regulatory domain. The bxd/pbx regulatory domain is set in the off state in parasegments (segments) anterior to PS6/A1 at the blastoderm stage and is repressed during the remainder of development by PcG-dependent silencing. In PS6/A1 (and more posterior segments), the bxd/pbx regulatory domain is set in the on state, activating the stage and tissue-specific enhancers in this domain. These stage- and tissue-specific enhancers activate the HS2 promoter in PS6/A1 and more posterior parasegments and transcription from this promoter through HS1 disrupts Fub-1 boundary function (Figure 8). This enables stage- and tissue-specific enhancers in bxd/pbx to activate Ubx expression in PS6/A1 and more posterior parasegments.
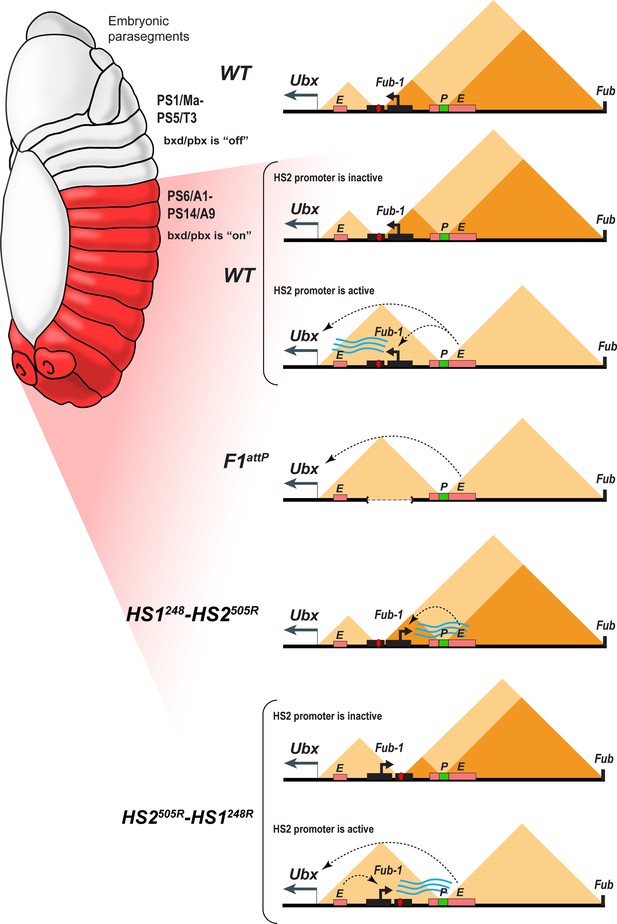
Model showing changes in TAD organization during Fub-1HS2 transcription.
Top: In WT, the 3D organization of the bxd/pbx regulatory domain transitions from one state to another when it activates Ubx expression (see also Mateo et al., 2019). In PS5 (T3) and more anterior parasegments, the bxd/pbx domain is encompassed in a large TAD that extends from Fub-1 to Fub (large triangle). Within this large TAD, there are two HDIC domains. One extends from Fub-1 to the bxd PRE, while the other extends from the bxd PRE to Fub. In PS6 and more posterior parasegments, the 3D organization of the domain is dynamic and depends upon whether the Fub-1 HS2 promoter is active or not. When the promoter is inactive (in between bursts), the Fub-1 boundary is expected to be functional and that TAD/HDIC organization would resemble that in more anterior parasegments. When the enhancers in bxd/pbx activate HS2, transcriptional read-through disrupts Fub-1 boundary activity generating a new TAD organization that links enhancers in bxd/pbx to the Ubx promoter. F1attP: deletion of the Fub-1 boundary eliminates the large Fub-1←→Fub TAD in all parasegments. In this case, an element upstream of the Ubx promoter functions to insulate abx/bx from bxd/pbx in PS5/T3. HS1248-HS2540R: In this replacement, HS2 is inverted so that the HS2 promoter is pointed away from HS1 toward the bxd/pbx regulatory domain. Though bxd/pbx activates the promoter in PS6/A1 and more posterior parasegments, Fub-1 boundary activity is not disrupted. HS2540R-HS1248R: In this replacement, Fub-1 is inverted. Though HS2 promoter activity in PS6 (A1) and more posterior parasegments is reduced in this configuration compared to WT, it is sufficient to disrupt Fub-1 boundary activity and enable bxd/pbx to regulate Ubx expression.
Several lines of evidence are consistent with this mechanism. When HS1 is used to replace the Fab-7 boundary, it functions like other generic fly boundaries and blocks crosstalk between the iab-6 and iab-7 initiators, but does not support boundary bypass. This blocking activity is also orientation independent. In contrast, neither HS2 alone nor the intact Fub-1 (HS1+HS2) element are able to block crosstalk between iab-6 and iab-7 and both replacements exhibit the same GOF transformations as the starting F7attP replacement platform. In the HS1+HS2 Fab-7 replacement experiments, the defects in Fub-1 blocking activity arise from transcriptional read-through from the HS2 promoter. This conclusion is supported by two lines of evidence. First, introducing the SV40 transcription termination polyadenylation element in between HS2 and HS1 partially rescues the blocking defect. Rescuing activity is not due to the increased distance between HS2 and HS1 as a SV40 element with mutations in the polyadenylation signal significantly compromising its rescuing activity. In addition, the SV40 termination element must be located between HS1 and HS2 as rescue is not observed when it is placed downstream of HS1. Second, when HS2 is inverted so that transcription proceeds away from HS1, boundary function is also restored.
A similar mechanism appears to regulate Fub-1 boundary activity in its endogenous context; however, in this case boundary activity is segmentally regulated. In PS5 and more anterior parasegments, the bxd/pbx regulatory domain is maintained in an ‘off’ state by a PcG-based mechanism. As a consequence, the Fub-1 HS2 promoter is inactive (Figure 8). In PS6 (A1) (and more posterior parasegments), the bxd/pbx domain is in the ‘on’ state and it activates transcription from the Fub-1 HS2 promoter. When HS2 is oriented so that transcription proceeds through HS1, boundary activity is abrogated in PS6/A1 and more posterior parasegments/segments (Figure 8). This enables enhancers in the bxd/pbx regulatory domain to activate Ubx transcription. Consistent with the parasegment-specific differences in the 3D topology imaging studies of fly embryos, Mateo et al. showed that the Ubx region of BX-C undergoes a reorganization in PS5/T3 and PS6/A1 (Mateo et al., 2019). This rearrangement would be blocked when HS2 is oriented away from HS1. In this case, boundary activity is retained and the stage and tissue enhancers in the bxd/pbx regulatory domain are unable to activate Ubx expression (Figure 8).
While it is clear that the Fub-1 boundary function is subject to negative and positive regulation by elements in the bxd/pbx domain, and that this is important for the proper specification of PS6/A1, there are a number of outstanding questions. One is whether transcription from the HS2 promoter is necessary to inactivate HS1 earlier in development. As described above, bxd lncRNA transcripts emanating from a promoter in pbx extend through both HS2 and HS1. If HS1 is sensitive to read-through from HS2, one would think that it would also be disrupted by transcriptional read-through of the bxd lncRNA. However, since Pease et al. found that transcription of the bxd lncRNA is not required for normal development, it would appear that this lncRNA is dispensable, at least when Fub-1 HS2 is present. While we do not know the pattern of Fub-1 or bxd lncRNAs expression after embryogenesis, the fact that reversing HS2 results in morphological defects in adults would argue that Fub-1 is likely expressed during the larval and pupal stages.
Another question is raised by the Micro-C contact pattern in WT and the Fub-1 deletion. In WT, Fub-1 and Fub define a TAD that encompasses (most of) bxd/pbx. Fub-1 also corresponds to a boundary for two smaller HDIC domains, one with a second endpoint close to the Ubx promoter and another with a second endpoint close to the bxd PRE. In the Fub-1 deletion, the proximal endpoint of the subTAD formed by Fub-1 and bxd PRE shifts to the Ubx promoter region, while the two HDIC domains collapse into one. If the function of HS2 is to inactivate HS1 in the posterior 2/3rds of the embryo, one would expect that this would disrupt the Fub-1 ←→ Fub TAD, much like the Fub-1 deletion. However, we observe a Fub-1 ←→ Fub TAD. Since the HS2 promoter should not be active in cells anterior to PS6/A1, it is possible that these cells are responsible for generating the Fub-1 ←→ Fub TAD seen in the Micro-C experiments. Alternatively (or in addition), the disruption in HS1 function might only occur during a burst of transcription (Figure 8). Recent studies have shown that transcription of most genes is not continuous but rather occurs in discrete bursts (Rodriguez and Larson, 2020; Tunnacliffe and Chubb, 2020). The frequency, duration, and amplitude of the burst can vary substantially. In some experimental systems, bursts can last several minutes or longer while the intervals between bursts may be only 10s of seconds (Bothma et al., 2014; Fukaya et al., 2016). For other genes, the intervals between bursts can be 30 min or more and the bursts may last only a minute or two (Alexander et al., 2019). If disruption of HS1 blocking activity is directly coupled to the act of transcription (as appears to be the case), then there may only be small windows of time in which the activity of the Fub-1 boundary is compromised and bxd/pbx enhancers can activate the Ubx promoter. In this case, the Fub-1 insulator would flip back and forth from an inactive and active state (Figure 8, see WT and HS2540R-HS1248R). Potentially consistent with a model along these lines, in stage 14 embryos, only a subset of cells in PS6 and more posterior parasegments have transcripts complementary to Fub-1HS2 (Figure 6).
A related issue would be the mechanism activating HS2 transcription when the Fub-1 boundary is reversed (the HS1248-HS2505R replacement) so that HS1 is interposed between HS2 and the bxd/pbx domain. One would have expected that HS1 would block the bxd/pbx enhancers from activating HS2 transcription. In this case, boundary function would be retained, resulting in an LOF transformation of PS6/A1 into PS5/T3 because it would prevent bxd/pbx from regulating Ubx. One likely possibility is that the putative enhancers in the region between the Ubx promoter and Fub-1 (see 2218S enhancer in Figure 1—figure supplement 1) are able to activate a sufficient level of HS2-dependent transcription to disrupt HS1 boundary function. This is supported by the pattern of expression of reporters inserted to either side of Fub-1 (Figure 3). An alternative/additional possibility is that the blocking activity of HS1 is not sufficient to completely suppress regulatory interactions between bxd/pbx and HS2. Potentially consistent with this idea is the finding that the orientation of the Fub-1 element in the Fab-7 replacements does not seem to be important. When HS2 is adjacent to iab-6, it inactivates the blocking activity of HS1. This would be the expected result since reporters in BX-C are activated when the regulatory domain in which they are inserted is turned on in the early embryo. In this case, iab-6 would drive HS2 expression in PS11(A6) cells and this would inactivate the blocking activity of HS1 in cell in this parasegment, giving a GOF transformation of PS11/A6. The unexpected result is that a GOF transformation of PS11/A6 into PS12/A7 is also observed when the Fub-1 replacement is inserted so that HS2 is next to iab-7. Since iab-7 is ‘off’ in PS11, this would imply that HS2 must be activated by the iab-6 regulatory domain. A similar scenario could be at play when Fub-1 is inserted in the opposite orientation in its endogenous location: HS1 blocks but does not completely eliminate interactions between the bxd/pbx enhancers and the HS2 promoter. This is probably not unexpected. While interposed boundaries significantly reduce enhancer:promoter contacts, they are not completely eliminated (Fukaya et al., 2016). In addition, once activated, transcription from HS2 through HS1 should weaken HS1 boundary activity and this in turn would facilitate further transcription.
Perhaps the most puzzling question is what function does Fub-1 play in BX-C regulation? Since there is no obvious GOF phenotype when Fub-1 is deleted, it differs from other boundaries in BX-C that have been analyzed in that would appear to be dispensable. On the other hand, when present, it must be inactivated in PS6/A1 (and more posterior parasegments) so that the bxd/pbx domain can regulate Ubx expression. Interestingly, like cis-acting elements for many other lncRNAs, both of the Fub-1 boundary subelements are conserved in the Sophorphora subgenus (Figure 1—figure supplement 3). It is also conserved in D. virilis, which is a member of the Drosophila subgenus. D. virilis differs from melanogaster in that the Ubx gene and its two regulatory domains, abx/bx and bxd/pbx, are separated from the abd-A and Abd-B genes. In spite of this rearrangement, the Fub-1 sequences are conserved. The fact that Fub-1 is present in distantly related species would argue that Fub-1 has an important function under some type of selective condition (that we have yet to discover) that is commonly encountered by a wide range of Drosophila species.
Materials and methods
Fly strains
Request a detailed protocolThe following stocks were used in these experiments: y[1] M{GFP[E.3xP3]=vas-Cas9.RFP-}ZH-2A w[1118] (BDSC_55821), w[1], ΦC31 (y+); TM6, Tb, Hm/Sb, w[1]; TM6, Tb, Hm/Sb, P{w[+mC]=ovoFLP.R}M1A, w[*] (BDSC_8727), y[1] w[67c23] P{y[+mDint2]=Crey}1b; D[*]/TM3, Sb[1] (BDSC_851) was used for all transgenesis. y[1] M{GFP[E.3xP3]=vas-Cas9.RFP-}ZH-2A w[1118] (BDSC_55821) was used for CRISPR/Cas9 genome editing. y[1] w[67c23] P{y[+mDint2]=Crey}1b; D[*]/TM3, Sb[1] (BDSC_851) was used for Cre/loxP recombination to remove DsRed from CRISPR-generated DsRed insertions. w[1]; TM6, Tb, Hm/Sb, P{w[+mC]=ovoFLP.R}M1A, w[*] (BDSC_8727) was used for Flp/frt recombination to remove GFP from ΦC31-mediated insertions. HS1248-HS2505R stock is weak and was grown on rich medium described by Backhaus et al., 1984. All other stocks were kept on standard fly cornmeal-molasses-yeast media.
Generation of F1attP by CRISPR-Cas9-induced homologous recombination
Request a detailed protocolFor generating double-stranded DNA donor template for homology-directed repair, we used pHD-DsRed vector (Addgene plasmid no. 51434). The final plasmid contains genetic elements in the following order: [bxd proximal arm]-[attP]-[loxP]-[3×P3-dsRed-SV40polyA]-[loxP]-[bxd distal arm] (Figure 1—figure supplement 1). Homology arms were PCR-amplified from the Bloomington Drosophila Stock Center line no. 55821 genomic DNA using the following primers: AGCTCTCGAGAGCGATGGAACCGTTTCTG and GTAGATCTCGGTTTACGATCGACTGGC for the proximal arm (1001 bp fragment), and TTGCGGCCGCATTTCTACGTTTATAAGCTGTTAATC and AAGCATGCCCACAATGGAGGCTGC for the distal arm (1002 bp fragment). Targets for Cas9 were selected using ‘CRISPR optimal target finder’—the program from O'Connor-Giles Laboratory. The following gRNA sequences, AGTCGATCGTAAACCGGAG and TTATAGACGTAGAAATGTA, were inserted into the pU6-BbsI-chiRNA vector (Addgene plasmid no. 45946). A mixture of the donor vector (500 ng/ml) and two gRNA vectors (100 ng/ml each) was injected into the embryos of y[1] M{GFP[E.3xP3]=vas-Cas9.RFP-}ZH-2A w[1118] (no. 55821 from the Bloomington Drosophila Stock Center). Injectees were grown to adulthood and crossed with w[1]; TM6/Sb line. Flies positive for dsRed signal in eyes were selected into a new separate line. The successful integration of the recombination plasmid was verified by PCR and corresponded to the removal of 1168 bp within the Fub-1 region (genome release R6.22: 3R:16,748,143.16,749,310).
Generation the replacement lines
Request a detailed protocolFor the F1attP replacement, the recombination plasmid was designed de novo and contains several genetic elements in the following order: [loxP]-[pl]-[frt]-[3P3-GFP]-[frt]-[attb] (Figure 1—figure supplement 1). All elements were assembled within the pBluescript SK vector. loxP site is located before polylinker [pl] and in combination with the second site, which is located in the platform, used for excision of DsRed marker gene and plasmid body. Two frt sites are used to excise GFP marker. DNA fragments used for the replacement experiments were generated by PCR amplification and verified by sequencing (presented in the Supplementary Methods).
The strategy of the Fab-7 replacement lines creation is described in detail in Wolle et al., 2015.
Adult and larval cuticle samples preparation
Request a detailed protocolThree-day adult male flies were collected in 1.5 ml tubes and stored in 70% ethanol at least 1 d. Then ethanol was replaced with 10% KOH and flies were heated at 70°C for 1 hr. After heating, flies were washed with dH2O two times and heated again in dH2O for 1 hr. After that, the digested flies were washed three times with 70% ethanol and stored in 70% ethanol. The abdomen cuticles were cut off from the rest of the fly using a fine tweezer and a needle of an insulin syringe and put in a droplet of glycerol on a microscope slide. Then the abdomens were cut longitudinally on the mid-dorsal side through all of the tergites with the syringe. Then the cuticles were flattened with a coverslip. HS1248-HS2505R third instars were used for larval cuticle preparation. This stock is homozygous sterile and was maintained on TM6B balancer. The TM6B balancer carries the Tb1 dominant mutation, which results in tubby larvae and pupae. Larvae without tubby phenotype were selected for cuticle preparation. Larval cuticle was prepared as described by Ingham, 1984. Photographs in the bright or dark field were taken with the Nikon SMZ18 stereomicroscope using Nikon DS-Ri2 digital camera, processed with Fiji bundle 2.0.0-rc-46.
Embryo immunostaining
Request a detailed protocolEmbryo immunostaining was performed as previously described (Deshpande et al., 1999). Primary antibodies were mouse monoclonal anti-Abd-B at 1:40 dilution (1A2E9, generated by S. Celniker, deposited to the Developmental Studies Hybridoma Bank), mouse monoclonal anti-DsRed at 1:50 dilution (Santa Cruz Biotechnology), and rabbit polyclonal anti-GFP at 1:500 dilution (Thermo Fisher Scientific). Secondary antibodies were goat anti-mouse Alexa Fluor 546 (Thermo Fisher Scientific) and goat anti-rabbit Alexa Fluor 488 (Thermo Fisher Scientific). Stained embryos were mounted with VECTASHIELD antifade mounting medium (Vector Laboratories). Images were acquired with a Leica STELLARIS 5 confocal microscope and processed using ImageJ 1.53f51 (Schindelin et al., 2012).
smFISH
Request a detailed protocolsmFISH was performed as previously described (Little et al., 2013). Quasar 670-conjugated Fub-1 sense and antisense probes were ordered from LGC Biosearch Technologies. Sequences are listed in Supplementary file 1b and c. Embryos were collected overnight and then dechorionated in Clorox, fixed in 4% paraformaldehyde, and devitellinized in methanol. The hybridization with the FISH probes was performed overnight at 37°C in hybridization buffer (10% dextran sulfate, 0.01% salmon sperm single-strand DNA, 1% vanadyl ribonucleoside, 0.2% bovine serum albumin, 4×SSC, 0.1% Tween-20, and 35% formamide). The resulting preparations were washed twice with wash buffer, 1 hr each, at 37°C and mounted in VECTASHIELD antifade mounting medium. Images were acquired with a Leica STELLARIS 5 confocal microscope with ×40 HC PL APO CS2 1.3 NA oil immersion objective. Image denoising was performed using NIS elements ‘Advanced denoising’ tool.
Micro-C library construction
Request a detailed protocolEmbryos were collected on yeasted apple juice plates in population cages. Plates were laid for 4 hr, incubated for 12 hr at 25℃, then subjected to fixation. Embryos were collected in nylon mesh sieves, dechorionated for 2 min in 3% sodium hypochlorite, rinsed with deionized water, and transferred to glass vials containing 5 ml PBST (0.1% Triton-X in PBS), 7.5 ml N-heptane, and 1.5 ml fresh 16% formaldehyde. Crosslinking was carried out at room temperature for exactly 15 min on an orbital shaker at 250 rpm, followed by addition of 3.7 ml 2 M Tris-HCl pH7.5 and shaking for 5 min to quench the reaction. Embryos were washed twice with 15 ml PBST and subjected to secondary crosslinking. Secondary crosslinking was done in 10 ml of freshly prepared 3 mM final DSG and ESG in PBST for 45 min at room temperature with passive mixing. Reaction was quenched by addition of 3.7 ml of 2 M Tris-HCl pH7.5 for 5 min, then washed twice with PBST. Embryos were snap-frozen and stored at –80℃ until library construction.
Micro-C libraries were prepared as previously described (Batut et al., 2022) with the following modification: we used 50 ul of 12–16 hr embryos, non-sorted for each biological replicate. 60U of MNase was used for each reaction to digest chromatin to 80% mononucleosome vs. 20% dinucleosome ratio. Libraries were barcoded, pooled, and subjected to paired-end sequencing on an Illumina Novaseq S1 100 nt Flowcell (read-length 50 bases per mate, 6-base index read).
Micro-C data processing
Request a detailed protocolMicro-C data for Drosophila melanogaster were aligned to a custom genome edited from the Berkeley Drosophila Genome Project (BDGP) Release 6 reference assembly (dos Santos et al., 2015) with BWA-MEM (Li and Durbin, 2009) using the parameters -S -P -5 -M. Briefly, the custom genome was designed to remove the visually obstructive ~5.6 kb DIVER_I-int repeat element between Fub-1 and Fub. The specific region deleted was the entire repeat element, chr3R: 16756620–16762262 from the dm6 reference FASTA file. The resultant BAM files were parsed, sorted, de-duplicated, filtered, and split with pairtools (https://github.com/open2c/pairtools; Goloborodko et al., 2023). We removed pairs where only half of the pair could be mapped or where the MAPQ score was less than three. The resultant files were indexed with pairix (https://github.com/4dn-dcic/pairix; Lee et al., 2021). The files from replicates were merged with pairtools before generating 100 bp contact matrices with Cooler (Abdennur and Mirny, 2020). Finally, balancing and mcool file generation was performed with Cooler’s zoomify tool. The mcool files were visualized using HiGlass (Kerpedjiev et al., 2018).
Appendix 1
Reagent type (species) or resource | Designation | Source or reference | Identifiers | Additional information |
---|---|---|---|---|
Genetic reagent (Drosophila melanogaster) | Cas9 | Bloomington Drosophila Stock Center | BDSC: 55821 | y[1] M{GFP[E.3xP3]=vas-Cas9.RFP-}ZH-2A w[1118] |
Genetic reagent (D. melanogaster) | ΦC31 | This lab | N/A | w[1], ΦC31 (y+); TM6, Tb, Hm/Sb |
Genetic reagent (D. melanogaster) | This lab | N/A | w[1]; TM6, Tb, Hm/Sb | |
Genetic reagent (D. melanogaster) | Fub-1attP | This paper | N/A | Available from Paul Schedl laboratory in Princeton university. Contact pschedl@princeton.edu |
Genetic reagent (D. melanogaster) | Fab-7attP50 | This lab | N/A | Available from Paul Schedl laboratory in Princeton university. Contact pschedl@princeton.edu |
Genetic reagent (D. melanogaster) | FLP | Bloomington Drosophila Stock Center | BDSC: 8727 | P{w[+mC]=ovoFLP.R}M1A, w[*] |
Genetic reagent (D. melanogaster) | Cre | Bloomington Drosophila Stock Center | BDSC: 851 | y[1] w[67c23] P{y[+mDint2]=Crey}1b; D[*]/TM3, Sb[1] |
Antibody | Anti- Abd-B (mouse monoclonal) | Developmental Studies Hybridoma Bank | 1A2E9 | IF(1:50) |
Antibody | Anti-Ubx (mouse monoclonal) | Developmental Studies Hybridoma | FP3.38 | IF(1:50) |
Antibody | Anti-DsRed antibody (mouse monoclonal) | Santa Cruz Biotechnology | sc-390909 | IF(1:50) |
Antibody | Anti-GFP antibody (rabbit polyclonal) | Thermo Fisher Scientific | A-11122 | IF(1:500) |
Antibody | Anti-mouse Alexa Fluor 546 (goat polyclonal) | Thermo Fisher Scientific | A-11030 | IF(1:500) |
Antibody | Anti-rabbit Alexa Fluor 488 (goat polyclonal) | Thermo Fisher Scientific | A-11008 | IF(1:500) |
Chemical compound, drug | Paraformaldehyde 20% solution, EM Grade | Electron Microscopy Sciences | 15713S | |
Chemical compound, drug | Formaldehyde, 16%, methanol free, Ultra Pure | Polysciences Inc | 18814-10 | |
Chemical compound, drug | Phosphate-buffered saline (10×) pH 7.4, RNase-free | Thermo Fisher | AM9624 | |
Chemical compound, drug | Tween 20 | Sigma | P1379 | |
Chemical compound, drug | Triton X-100 | Bio-Rad | 161-0407 | |
Chemical compound, drug | Tris base | Sigma | 11814273001 | |
Chemical compound, drug | Methanol | Fisher Chemical | 203403 | |
Chemical compound, drug | SSC, 20× | Thermo Fisher Scientific | 15557044 | |
Chemical compound, drug | Formamide | Thermo Fisher Scientific | 17899 | |
Chemical compound, drug | Dextran sulfate | Sigma | D8906 | |
Chemical compound, drug | Salmon Sperm DNA | Thermo Fisher Scientific | AM9680 | |
Chemical compound, drug | Ribonucleoside Vanadyl Complex | NEB | S1402S | |
Chemical compound, drug | Nuclease-free BSA | Sigma | 126609 | |
Chemical compound, drug | Triethylammonium acetate | Sigma | 625718 | |
Chemical compound, drug | dGTP (100 MM) | VWR | 76510-208 | |
Chemical compound, drug | dTTP (100 MM) | VWR | 76510-224 | |
Chemical compound, drug | Lonza NuSieve 3:1 Agarose | Thermo Fisher Scientific | BMA50090 | |
Chemical compound, drug | T4 DNA ligase | NEB | M0202L | |
Chemical compound, drug | Biotin-11-dCTP | Jen Bioscience | NU-809-BIOX | |
Chemical compound, drug | Biotin-14-dATP | Jen Bioscience | NU-835-BIO14 | |
Chemical compound, drug | Qubit dsDNA HS Assay Kit | Life Technologies Corporation | Q32851 | |
Chemical compound, drug | Phase Lock Gel, QuantaBio - 2302830, Phase Lock Gel Heavy | VMR | 10847-802 | |
Chemical compound, drug | NEBNext Ultra II DNA Library Prep Kit for Illumina | NEB | E7645S | |
Chemical compound, drug | Ampure Xp 5 ml Kit | Thermo Fisher Scientific | NC9959336 | |
Chemical compound, drug | Hifi Hotstart Ready Mix | Thermo Fisher Scientific | 501965217 | |
Chemical compound, drug | Dynabeads MyOne Streptavidin C1 | Life Technologies Corporation | 65001 | |
Chemical compound, drug | cOmplete, EDTA-free Protease Inhibitor Cocktail | Sigma | 11873580001 | |
Chemical compound, drug | N,N-Dimethylformamide | Sigma | 227056 | |
Chemical compound, drug | Potassium acetate solution | Sigma | 95843 | |
Chemical compound, drug | DSG (disuccinimidyl glutarate) | Thermo Fisher Scientific | PI20593 | |
Chemical compound, drug | T4 Polynucleotide Kinase – 500 units | NEB | M0201S | |
Chemical compound, drug | DNA Polymerase I, Large (Klenow) Fragment – 1000 units | NEB | M0210L | |
Chemical compound, drug | End-it DNA End Repair Kit | Thermo Fisher Scientific | NC0105678 | |
Chemical compound, drug | Proteinase K recomb. 100 mg | Sigma | 3115879001 | |
Chemical compound, drug | Nuclease Micrococcal (s7) | Thermo Fisher Scientific | NC9391488 | |
Chemical compound, drug | EGS (ethylene glycol bis(succinimidyl succinate)) | Thermo Fisher Scientific | PI21565 | |
Sequence-based reagent | Fub-1 sense probe set | Biosearch Technologies | N/A | |
Sequence-based reagent | Fub-1 antisense probe set | Biosearch Technologies | N/A | |
Software, algorithm | Fiji (ImageJ) | Schindelin et al., 2012 | fiji.sc | |
Software, algorithm | NIS element | Nikon | https://www.microscope.healthcare.nikon.com/products/software/nis-elements/ | |
Software, algorithm | GraphPad Prism 8 | GraphPad Software | https://www.graphpad.com | |
Software, algorithm | HiGlass | Kerpedjiev et al., 2018 | http://higlass.io/ | |
Software, algorithm | bwa | Li and Durbin, 2009 | https://bio-bwa.sourceforge.net/ | |
Software, algorithm | samtools | Github/open source | https://samtools.github.io | |
Software, algorithm | pairsamtools | Github/open source; Goloborodko et al., 2023 | https://github.com/open2c/pairtools | |
Software, algorithm | pairix | Github/open source; Lee et al., 2021 | https://github.com/4dn-dcic/pairix | |
Software, algorithm | cooler | Abdennur et al., 2023 | https://github.com/open2c/cooler | |
Software, algorithm | Miniconda | Anaconda | https://docs.conda.io/en/latest/miniconda.html | |
Software, algorithm | Snakemake | Github/open source | https://snakemake.github.io |
Data availability
Sequencing data have been deposited in GEO under accession code GSE217005. The data can be accessed using the following secure token sxyxomkyjlgxvmj.
-
NCBI Gene Expression OmnibusID GSE217005. The insulating activity of the Drosophila BX-C chromatin boundary Fub-1 is parasegmentally regulated by lncRNA read-through.
References
-
A semi-synthetic, general-purpose medium for Drosophila melanogasterDrosophila Information Service 60:210–212.
-
The Variable CTCF Site from Drosophila melanogaster Ubx Gene is Redundant and Has no Insulator ActivityDoklady Biochemistry and Biophysics 505:173–175.https://doi.org/10.1134/S1607672922040044
-
A lifelong duty: how Xist maintains the inactive X chromosomeCurrent Opinion in Genetics & Development 75:101927.https://doi.org/10.1016/j.gde.2022.101927
-
The boundary paradox in the Bithorax complexMechanisms of Development 138 Pt 2:122–132.https://doi.org/10.1016/j.mod.2015.07.002
-
Mechanisms of Interaction between Enhancers and Promoters in Three Drosophila Model SystemsInternational Journal of Molecular Sciences 24:2855.https://doi.org/10.3390/ijms24032855
-
Author Correction: Chromatin-associated RNAs as facilitators of functional genomic interactionsNature Reviews. Genetics 20:503–519.https://doi.org/10.1038/s41576-019-0155-x
-
Structural modularity of the XIST ribonucleoprotein complexNature Communications 11:6163.https://doi.org/10.1038/s41467-020-20040-3
-
The ABC of the BX-C: the bithorax complex explainedDevelopment 133:1413–1422.https://doi.org/10.1242/dev.02323
-
Emerging Functions of lncRNA Loci beyond the Transcript ItselfInternational Journal of Molecular Sciences 23:6258.https://doi.org/10.3390/ijms23116258
-
Transcription in living cells: molecular mechanisms of burstingAnnual Review of Biochemistry 89:189–212.https://doi.org/10.1146/annurev-biochem-011520-105250
-
Fiji: an open-source platform for biological-image analysisNature Methods 9:676–682.https://doi.org/10.1038/nmeth.2019
-
Gene regulation by long non-coding RNAs and its biological functionsNature Reviews Molecular Cell Biology 22:96–118.https://doi.org/10.1038/s41580-020-00315-9
-
What Is a Transcriptional Burst?Trends in Genetics 36:288–297.https://doi.org/10.1016/j.tig.2020.01.003
-
Functional Requirements for Fab-7 Boundary Activity in the Bithorax ComplexMolecular and Cellular Biology 35:3739–3752.https://doi.org/10.1128/MCB.00456-15
Article and author information
Author details
Funding
National Institute of General Medical Sciences (R35 GM126975)
- Paul Schedl
National Institute of General Medical Sciences (R01 GM118147)
- Michael Levine
Russian Science Foundation (20-14-00201)
- Yulii V Shidlovskii
Russian Science Foundation (19-14-00103)
- Pavel Georgiev
Ministry of Science and Higher Education of the Russian Federation (075-15-2019-1661)
- Pavel Georgiev
The funders had no role in study design, data collection and interpretation, or the decision to submit the work for publication.
Acknowledgements
We are grateful to the Center for Precision Genome Editing and Genetic Technologies for Biomedicine of IGB RAS and the Core Facilities Center of IGB RAS for providing research equipment. We thank Tatyana Smirnova for technical assistance and advice on smFISH data acquisition and analysis. We thank Girish Deshpande, Tsutomu Aoki, Olga Kyrchanova, and Oleg Bylino for insightful discussions throughout the course of this work. This work was supported by R35 GM126975 to PS and R01 GM118147 to ML. Part of this work (cloning) was supported by the Russian Science Foundation, Project No. 20-14-00201 (to YS). Part of this work (transgenesis) was supported by the Russian Science Foundation (19-14-00103) and grant no. 075-15-2019-1661 from the Ministry of Science and Higher Education of the Russian Federation (immunohistochemistry).
Copyright
© 2023, Ibragimov et al.
This article is distributed under the terms of the Creative Commons Attribution License, which permits unrestricted use and redistribution provided that the original author and source are credited.
Metrics
-
- 1,105
- views
-
- 132
- downloads
-
- 12
- citations
Views, downloads and citations are aggregated across all versions of this paper published by eLife.